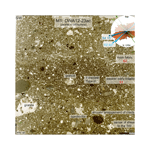
Micromorphology and clast microfabrics of subglacial traction tills at the sea cliff Dwasieden: evidence of polyphase syn- and post-depositional deformation
Johannes Brumme
Emrys Phillips
A detailed thin-section-based micromorphological and microstructural study of the glacial diamicts exposed at the sea cliff of Dwasieden (M1, M2, M2) has revealed that all units can be related, in their entirety or in several parts, to subglacial conditions during the repeated readvance of the Scandinavian Ice Sheet. These readvances are characterised by polyphase deformation of the diamicts resulting in the development of ductile and brittle structures and localised water-escape structures. Subsequent alteration under periglacial conditions has been documented for the chalk and till units M1 and M2.
- Article
(20444 KB) - Full-text XML
- BibTeX
- EndNote
In this contribution, the Pleistocene record exposed at the sea cliff of Dwasieden near Sassnitz (54∘30′0.44′′ N, 13∘36′46.09′′ E) is explored, focusing on the origin of the glacial diamicts. A detailed micromorphology study (Brumme, 2015) has allowed the unravelling of the complex depositional and deformation histories recorded by this sedimentary sequence with respect to ice flow across Jasmund. The analysis of the microstructures (Phillips et al., 2011; Brumme, 2015) observed in thin sections of the diamictons has revealed a chronology of deformational events which can be interpreted in the context of syn-depositional till-forming processes and post-depositional modification (e.g. permafrost, glacitectonic imbrication). More broadly, the geometry and cross-cutting relationships of the depositional units and glacitectonic (micro-)structures documented at this locality and other outcrops have enabled the construction of a tectono-stratigraphic model for the evolution of the Jasmund area during the Weichselian.
The cliff section at Dwasieden exposes the late Cretaceous (Maastrichtian) to late Pleistocene (Weichselian) record forming part of the Jasmund Glacitectonic Complex (Gehrmann et al., 2019). The site is located in a proximal position within the southern structural sub-complex (Gehrmann and Harding, 2018; Gehrmann, 2018) and is oriented parallel to strike of this imbricate thrust stack. The exposed succession largely occurs within a single imbricate thrust slice and shows sub-horizontal stratification. However, at the southwestern end of the cliff, the steeply dipping chalk of the next, more proximal thrust sheet can be clearly seen thrust onto the much younger Pleistocene sediments. Previous work on the stratigraphy, sedimentary environmental conditions and glacitectonic deformation observed at Dwasieden has been published by Ludwig (1954/1955), Strahl (1988), Kanter (1989), Panzig (1989), Krienke (2004), Ludwig and Panzig (2010), Beiche (2014), Brumme (2015), Kielczynski (2016) and Pisarska-Jamroży et al. (2018a, b).
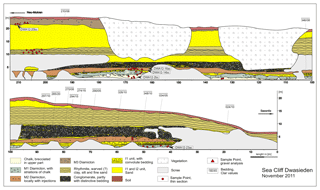
Figure 1Simplified geological cliff section of Dwasieden southwest of Sassnitz (modified from Brumme, 2015).
The Pleistocene sedimentary sequence exposed at Dwasieden is representative of Jasmund (see Kenzler et al., 2019). The late Cretaceous chalk observed at the base of the cliff is overlain by three Pleistocene till units (M1, M2 and M3), which are interbedded by gravels, sands and silt-clay rhythmites (units I1 and I2) (Fig. 1). Although most of the lithostratigraphic boundaries are related to hiatuses resulting from breaks in sedimentation and/or erosion, two of these boundaries represent major regional unconformities: (i) an erosional disconformity at the base of the M1 till, which represents a major break of ∼65 Myr between the latest Cretaceous and onset of Pleistocene sedimentation; and (ii) an angular unconformity at the base of the M3 till, which is formed during the late Weichselian and truncates the thrust sheets of the Jasmund Glacitectonic Complex.
The stratigraphic context aided by optically stimulated luminescence (OSL) dating indicates that the Pleistocene succession was mainly laid down during the Weichselian advance of the Scandinavian Ice Sheet. Pisarska-Jamroży et al. (2018a) concluded that the upper part of the I1 unit must have been deposited between 22.7±1.9 and 19.0±2.3 ka, i.e. shortly before the first late Weichselian ice advance reached the Jasmund peninsula (see Kenzler et al., 2017; Kenzler et al., 2019). Thus, the M2 till that directly overlies the I1 sedimentary record is thought to represent the Brandenburgian advance phase of the ice sheet during the Last Glacial Maximum (LGM). The I2 sedimentary record represents a short phase of ice retreat during post-LGM oscillations of the ice front around 17.9±1.8 ka, which is derived form an OSL age of a thin aeolian sand layer at the base of a glacilacustrine sand-silt-clay rhythmite deposited during the subsequent ice advance, most likely the Pomeranian phase of the Weichselian glaciation (cf. Kenzler et al., 2017). This ice advance is interpreted as having caused the glacitectonic imbrication of Jasmund and subsequently deposition of the M3 till.
Chalk. The uppermost part of the chalk is brecciated, comprising chalk clasts and randomly distributed clusters of flints within a soft chalky matrix. Large erratic boulders of crystalline rocks are found enclosed within the chalk up to 1.5 m below its upper boundary. Furthermore the chalk also contains isolated streaks of diamict material similar to the overlying M1 till. The top of the chalk is highly irregular and locally deformed by complex disharmonic folds and flame-like structures that extend into the overlying M1 diamicton.
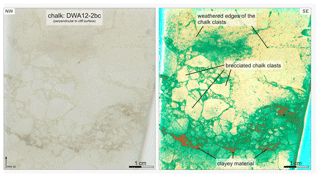
Figure 2High-resolution scan and false-colour image of thin section DWA12-2bc taken from the brecciated uppermost part of the chalk at the Dwasieden cliff section (from Brumme, 2015).
Micromorphology. In this section (Fig. 2) the highly brecciated chalk consists of angular to subangular clasts, which locally show diffuse and/or corresponding boundaries, set within a micritic carbonate or chalk-rich siliciclastic matrix containing lithic fragments of magmatic and metamorphic rocks similar to those observed within the overlying till. Large isolated quartz grains were locally observed within both the matrix of the chalk breccia and even within the chalk clasts. Evidence of the flow of water through the breccia and involution of fine-grained material from the overlying M1 till into the altered chalk bedrock is provided by the presence of irregular pockets and veins of clay (cutan) which possess a well-developed unistrial plasmic fabric.
M1. The clast-rich, blue-grey M1 diamicton is approximately 1.5 m thick and contains stringers or lenses of medium sand to fine gravel. Red-brown Fe-stained patches indicate that this diamict has undergone at least some secondary alteration. The base of the till is deformed by flame structures and overturned drag folds, which show a distinct vergence towards the SW. Macrofabric analysis carried out on pebble- and boulder-sized clasts within the M1 till reveals that their ab planes typically dip towards the NE. Locally developed clusters of subvertically oriented clasts are also present. Large boulders locally occur on the M1 surface or are partially embedded within the diamicton.
Micromorphology. Thin sections of the M1 diamicton reveal that crushed grains are very common and that deformation structures include turbate structures (ductile) and micro-faults (brittle). Water-escape structures are also common and contain fine, micritic carbonate translocated from the underlying chalk into the diamicton.
Detailed mapping of the clast microfabrics defined by the preferential shape alignment of detrital (skeleton) grains (Phillips et al., 2011) in thin sections oriented parallel to the ice flow (i.e. towards the SW) reveals the presence of up to four microfabrics formed in response to subglacial deformation (labelled S1 to S4 on Fig. 3); the dominant fabrics are a planar S1 microfabric which dips at a low to moderate angle towards the NE (i.e. up-ice) and a linear S2 fabric plunging at a moderate angle towards the SW (i.e. down-ice) (Brumme, 2015).
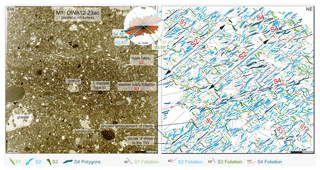
Figure 3High-resolution scan and microstructural map of thin section DWA12-23ac taken from the upper part of the M1 diamicton at the Dwasieden cliff section (modified from Brumme, 2015). The rose diagram shows the maxima of grain long-axis orientation. The earliest clast microfabric (S1) represents a discontinuous and rough foliation defined by relatively short domains, which dip at ∼40∘ to the NE. The S1 domains typically exhibit a distinctive sigmoidal geometry which records an apparent sinistral sense of shear. This early foliation is cut by a younger clast microfabric (S2), which is more pervasively developed and dips at ∼40∘ towards the SW. The disjunctive and more planar S2 fabric is characterised by relatively continuous microlithons. The spacing of S2 domains varies across the thin section, indicating that deformation during the imposition of this fabric was heterogeneous, with the earlier S1 fabric being preserved within the wider S2 microlithons. S1 and S2 are locally cut by a weak S3 fabric, which dips at to the NE and is defined by relatively short, irregular domains. In contrast to the earlier developed fabrics (S1 to S3), the locally well developed S4 clast microfabric occurs in discrete bands, which dip at towards the SW. This foliation clearly cross-cuts S1, S2 and S3 and is the youngest fabric.
I1. The I1 unit is characterised by a sequence of horizontally interbedded fine sands, silts and clays. The unit also contains an irregular gravel layer (20 m long, 0.2 m thick) comprising clusters of boulder- to pebble-sized clasts. The gravel is occasionally covered by a thin drape of sand. Isolated outsized elongate boulders also occur elsewhere within the I1 unit in some cases orientated almost perpendicular to bedding within the host sediment. Sediments around these outsized clasts are deformed, showing down-warped lamination and lateral truncation.
In addition, soft-sediment deformation structures occur at three levels within the I1 unit. The well-laminated sands and silts display extremely well developed convolute bedding, load casts, ball-and-pillow structures, pseudo-nodules and water-escape structures. An ice-wedge cast extends from the top of the lowermost soft-sediment deformation structure down to the underlying M1 till.
M2. The M2 till is a massive, clast-poor deposit with sharp erosional lower and upper boundaries. At its base the diamicton includes a highly deformed glacitectonic mélange containing disrupted lenses (intraclasts) of well-bedded sand. These intraclasts are lithologically similar to, and therefore derived from, the underlying I1 unit. Furthermore, there are stringers of sand incorporated into the diamicton as a result of the attenuation of a drag folds which deform at the M1–I1 boundary. These sand stringers are deformed by SW-verging folds and sigmoidal strain markers recording a SE-directed sense of shear.
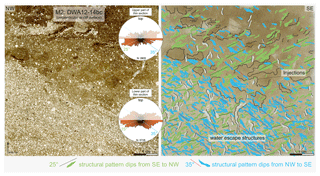
Figure 4High-resolution scan and microstructural map of thin section DWA12-14bc, taken from the base of the M2 diamicton at the Dwasieden cliff section (modified from Brumme, 2015). The rose diagram shows the maxima of grain long-axis orientation. Note water-escape structures and injections into the upper part. Distinctly oriented microfabrics mainly characterise the more-coarse-grained lower part. The earliest clast microfabric (S1) represents a discontinuous foliation defined by relatively short domains, which show an apparent dip between ∼15 (lower part) and (upper part) to the NW. A younger clast microfabric (S2), which is more pervasively developed, shows an apparent dip at towards the SE. Steeply inclined grains form a third clast microfabric (S3), which is related to water-escape structures and cross-cuts the older S1 and S2.
Micromorphology. In thin sections the clay-rich areas of the till possess a weakly to well-developed omnisepic plasmic fabric. Discrete shear zones which cross-cut the diamicton are marked by unistrial plasmic fabrics, while matrix-rich coating around larger grains show weak skelsepic plasmic fabrics. Microstructural mapping reveals the till possesses at least four microfabrics (S1, S2, S3, S4) (Figs. 4, 5), which is comparable to the underlying M1 diamicton and is therefore once again consistent with subglacial deformation imposed by ice advancing from the NE.
I2. The heterogeneous sedimentary unit I2 consists of interbedded gravels, sand-silt-clay rhythmites and well-sorted sands. The lower subunit (3–4 m thick) comprises crudely stratified boulder-rich sandy gravels which rest directly upon the M2 diamicton. In the northeastern part of the cliff section, the gravels are overlain by a subunit (2–5 m thick) of rhythmically bedded (? varved) fine-grained sands, silts and clays, which regularly include clay-intraclast layers (Beiche, 2014). Locally, the rhythmites are folded and contain outsized clasts (dropstones). Towards the southwest, they interfinger with a subunit (5–25 m) of cross-laminated and cross-bedded sand, which also possess climbing-ripple cross lamination, channelised cross bedding, upper plane bed lamination, antidunes and humpback dunes (Kielczynski, 2016). Furthermore, gravity-induced structures produced by sediment creep, slumping and sliding have been documented. In addition, outsized clasts (dropstones) occur.
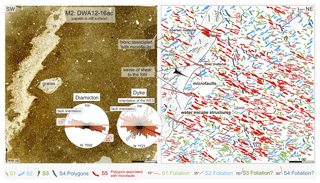
Figure 5High-resolution scan and microstructural map of thin section DWA12-16ac, taken from the centre of the M2 diamicton at the Dwasieden cliff section (modified from Brumme, 2015). The rose diagram shows the maxima of grain long-axis orientation. Note the district set of five clast microfabric domains, which display strain hardening. The oldest S1 dips at to the NE, while the main S2 dips at to the SW. Both S1 and S2 are cut by younger clast microfabric domains, including the straight, parallel-oriented S5, which dips at to the NE. The latter parallels and corresponds with reverse faults that dissect the subvertical dyke structure (sinistral sense of shear). Note high percentage of steeply inclined grain long axes within the dyke (see lower rose diagram).
M3. The brown M3 diamicton (2.5–3 m thick) is a sandy deposit rich in chalk stringers, which also contains a number of gravel lenses or intraclasts (10–20 cm long). This till also possesses a well-developed sub-horizontal set of joints.
Micromorphology. The common occurrence of crushed grains is obvious in thin sections. A district succession of microfabric domains has been documented by microstructural mapping (S1, S2, S3, S4) (Fig. 6).
3.1 Periglacial versus subglacial soft-sediment deformation of the chalk and the M1 diamicton
The brecciated texture of the chalk is interpreted as resulting from a near-surface (in situ) fragmentation of the Pleistocene bedrock due to frost weathering by seasonal freeze and thaw associated with the development of an active layer above permafrost. Murton (1996) describes a similar disruption from the chalk of southeast England. The folded upper boundary provides evidence that the base of M1 and the top of the underlying chalk has undergone contemporaneous soft-sediment deformation. This may be caused by ductile subglacial deformation during ice advance from the NE and/or involutions caused by periglacial activity.
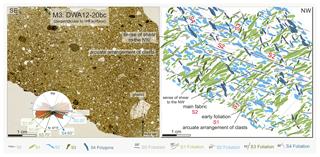
Figure 6High-resolution scan and microstructural map of thin section DWA12-20bc (oriented perpendicular to the cliff), taken from the M3 diamicton at the Dwasieden cliff section (modified from Brumme, 2015). The structural rose diagram shows the maxima of grain long-axis orientation. There are two main microfabrics: the older S1 is represented by long and anastomosing domains that dip between ∼55 and to the SE. Sigmoidal polygons indicate a dextral sense of shear. A younger, very distinct S2 fabric defined by parallel-oriented domains dips at to the NW.
3.2 Origin of the M1 diamicton: subglacial traction till or reworked periglacial deposit?
Shear-sense indicators (drag folds, bladed boulders) have been used to reconstruct a SW-directed ice flow during deposition of the M1 till (Brumme, 2015). Macroscopic and micromorphological features give evidence of both ductile and brittle deformation within the M1 till, which is a well-known feature in till successions (e.g. Larsen et al., 2006). The common occurrence of crushed grains within the M1 diamicton is indicative of subglacial deformation (van der Meer, 1993), although those features were also described from mass flow deposits (Lachniet et al., 2001). Clear evidence of subglacial deformation, however, reveals the presence of a pervasive distinctive set of clast microfabrics within the M1 diamicton (Brumme, 2015), documenting a polyphase deformation at the glacier bed (e.g. van der Meer, 1993; Phillips et al., 2018). They record a gradual shift from ductile (C'-type shear bands related to S1 and S2) to brittle deformation due to a decrease in the water content (localised S4 shear bands), which is known as strain hardening (Hiemstra and Rijsdijk, 2003).
The clusters of vertically aligned cobble- and boulder-sized clasts (erratics) within glacial sediments typically indicate that the sediment has subsequently undergone periglacial activity; the clasts are displaced/rotated due to frost heave. This feature together with the involutions, partial mixing of M1 with the chalk, and the alteration of the chalk bedrock clearly reveal the impact of permafrost. The presence of water-escape structures are indications for an initial high-water content within the M1 till.
3.3 The I1 sediment: evidence of periglacial activity
The fine-grained I1 sediments are regarded as having accumulated in a glacilacustrine environment close to the ice margin (Ludwig, 1954/55; Ludwig and Panzig, 2010; Brumme, 2015). The interbedded gravel layer and the outsized clasts represent ice-rafted debris formed by dumpstones and dropstones from drifting ice bergs (Pisarska-Jamroży et al., 2018b).
The soft-sediment deformation structures that occur together with an ice-wedge cast in the lower part (SSDS-1) are thought to provide evidence for periglacial activity during and after the accumulation of the I1 unit probably in response to annual (seasonal) freeze–thaw (Brumme, 2015). However, soft-sediment deformation in the upper part (SSDS-1, 2) have been interpreted by Pisarska-Jamroży et al. (2018a) as seismites potentially providing evidence for glacio-isostatically induced crustal faulting in front of the advancing Scandinavian Ice Sheet during the late Weichselian (Last Glacial Maximum, MIS-2).
3.4 Origin of the M2 diamicton: a subglacial mélange formed as a result of the deformation of soft permafrost
The macroscopic features are indicative of a glacitectonite at the base of the M2, which includes incorporated or plucked intraclasts and highly attenuated, folded and boudinaged laminae of the underlying sediment. The laminae are vertically stacked in the till and thereby represent tectonic slices produced during sequential phases of till accumulation. The preservation of primary lamination within the intraclasts is attributed to deformation of “warm” permafrost at temperatures slightly below the pressure-melting point, when pore ice cemented the intraclasts as rigid bodies (see Waller et al., 2011). Drag folds and sigmoidal strain markers indicate that the M2 till was laid down by ice advancing from the NE (Brumme, 2015).
3.5 Fabric development and dewatering during the deposition of the M2 diamicton: evidence for subglacial deformation of a water-saturated traction till
Micromorphological investigations show that both the shear-structured glacitectonic melange at the base and the homogenised-massive main M2 diamicton can be classified as a subglacial traction till (see Evans et al., 2006). Several thin sections from the M2 (e.g. Figs. 4, 5) reveal that there are two dominant gently dipping clast microfabrics omnipresent throughout this diamicton, forming a consistent pattern of short and discontinuous S1 domains (dip to the NNE), intersected by subparallel to anastomosing S2 domains (dip to the SSW, i.e. corresponding to the inferred ice flow). These microfabrics display preferred grain orientation as a result of shear at the glacier bed under ductile conditions (Brumme, 2015).
These early formed fabrics are cut by a subvertical to steeply inclined (? S3 and) S4 fabric (Figs. 4, 5) which is thought to have developed during dewatering of this water-saturated diamicton. The upward escape of the porewater through the sediment would have been concentrated into narrow zones or fluid pathways. Within these zones the finer-grained clasts were re-orientated by the escaping porewater.
Continuing deformation resulted in the imposition of the locally preserved S5 fabric (Fig. 5) that occurs coplanar to a set of small-scale faults offsetting a steeply inclined, sand-filled hydrofracture, which cuts the M2 diamicton.
Interestingly, the sense of displacement on these NE-dipping faults is consistent with the shear sense recorded by the sigmoidal, S–C-like fabric geometries of the S1 and S2 domains, with both sets of kinematic indicators indicating a sense of shear towards the SW/SSW (i.e. in response to an ice advance from the NE/NNE). This microstructural evidence can be used to suggest that the imposition of S1 and S2 under ductile conditions and the subsequent brittle faulting associated with the S5 imprint occurred as a result of the same overall stress regime. The deformation history recorded by M2 can therefore be considered to be polyphase, consisting of an early phase of more ductile deformation and the imposition of S1 and S2 when the M2 diamicton was probably water saturated, followed by more brittle deformation (due to water escape) leading to localised faulting and S5 fabric development. Micromorphological evidence clearly indicates that the earlier ductile phase of deformation (S1, S2) and later brittle phase were separated by a phase of dewatering and hydrofracturing, resulting in the formation of S3 and injection of the sand-filled veins (see Figs. 4, 5). Dewatering during the proposed progressive deformation events would have resulted in a change in the rheological properties and strain hardening of the diamicton.
3.6 Evidence for hydrofracturing and sediment injection during deposition of the M2
Macro- and microscale observations reveal that the M2 diamicton is cut by a number of sand-filled veins interpreted as hydrofractures. Microfabrics from the lower part (Fig. 4) indicate that liquefaction and injection of the underlying I1 sediments into M2 occurred penecontemporaneous to the deposition of the deformation till. Structurally higher within the M2 diamicton, the sand-filled veins are connected with larger, irregular patches of sand. Liquefaction and injection of the sand into M2 clearly indicate that the I1 was locally water saturated and, at least in part, unfrozen. Loading by the ice would have led to over-pressurised porewater within the locally melting permafrozen sand, leading to liquefaction, hydrofracturing of the basal part of the overlying diamicton and injection of the sand upward into the M2 as it was being deposited.
Within the upper, homogeneous part of M2, more brittle fabrics have been formed due to the ongoing dewatering during the advanced emplacement and consolidation of the diamicton. Cross-cutting relationships show that the formation of hydrofractures post-dates imposition of the ductile S1 and S2 clast microfabrics but occurred prior to the imposition of S5 and the associated faulting (Fig. 5).
3.7 The I2 sedimentary sequence: evidence for glacifluvial and glacilacustrine sedimentation
The I2 unit was deposited in glacifluvial and glacilacustrine environments at the ice margin (Ludwig, 1954/1955; Ludwig and Panzig, 2010; Beiche, 2014; Brumme, 2015). The heterogeneous interbedded facies has been interpreted as a glacial fan-delta complex developed at the margin of retreating ice sheet (Kielczynski, 2016). The crudely stratified gravels were deposited by debris flows and migrating transverse bed forms in a glacifluvial setting. In contrast, the rhythmically bedded sand-silt-clay couplets record sedimentation in a proglacial lake with temporal ice contact, as indicated by local folding, dropstones and characteristics of the varved lamination. The embedded in situ clay-intraclast layers are probably formed by annual freezing and thawing of the lake water and the lake bottom (Beiche, 2014). The varied sedimentary structures preserved within the thick sand subunit document rapidly changing flow conditions across the fan-delta cone (Kielczynski, 2016). The widely distributed gravity-induced folding structures may result from the steep depositional slope, liquefaction and even current drag.
3.8 Origin of the M3 diamicton: evidence of subglacial entrainment of chalk bedrock and formation of a traction till with polyphase deformation
The prominent chalk stringers within the M3 till provides clear evidence that the Cretaceous bedrock was exposed and prone to erosion during the advance of the Scandinavian Ice Sheet responsible for large-scale glacitectonism on the Jasmund peninsula and subsequent deposition of this subglacial till. The shape of sheared gravel intraclasts indicates a sense of shear towards the northwest. Macroscopic grain long-axis measurements show a preferred alignment of grain a axes to the NW, dipping to the SE (up-glacier). Microscopically, there is clear evidence of both ductile and brittle deformation during formation of the M3 diamicton (fabrics S1, S2, S3, S4), allowing a classification as traction till.
All till samples show evidence of polyphase deformation. There is predominantly pervasive ductile deformation in all thin sections, indicating a high initial water content under syndepositional conditions. Water-escape structures are typically related to late-stage ductile deformation. More local evidence of brittle deformation is indicative shear zones formed during later phases of deformation (syn- and post-depositional). The thin sections presented and discussed here are a small example of the potential when studying polyphase deformation at a microscale by means of a 2-D and 3-D microstructural mapping approach (see Phillips et al., 2011; Brumme, 2015).
All underlying data are published in the figures of this article. Thin sections are stored in the thin-section archive of the Institute of Geography and Geology at the University of Greifswald.
JB carried out field work and processed, measured and analysed the samples. JB wrote the first draft of the manuscript and developed most of the illustrations. HH designed the project, secured funding and rewrote part of the manuscript. HH and EP supervised JB during his PhD. All authors contributed to the discussion and interpretation of the presented research results.
The authors declares that there is no conflict of interest.
The study has been financially supported by the DFG (German Research Foundation, projects HU 804/6-1, FR 877/16-1). We thank Sylvia Weinert (University of Greifswald) for careful preparation of thin sections. The constructive comments of Karsten Schütze and an unknown reviewer substantially improved our paper. We acknowledge support for the article processing charge from the DFG (no. 393148499) and the Open Access Publication Fund of the University of Greifswald.
This research has been supported by the DFG (German Research Foundation, grant no. 393148499 and projects HU 804/6-1, FR 877/16-1) and the Open Access Publication Fund of the University of Greifswald.
Beiche, T.: Microfacies and depositional environment of Weichselian silt-clay rhythmites at the coastal cliff of Dwasieden (Jasmund, Rügen, Pomerania), Master-Thesis, University of Greifswald, 1–58, Greifswald, 2014.
Brumme, J.: Three-dimensional microfabric analyses of Pleistocene tills from the cliff section Dwasieden on Rügen (Baltic Sea Coast): Micromorphological evidence for subglacial polyphase deformation, Doctoral thesis, University of Greifswald, 1–250, 2015.
Evans, D. J. A., Phillips, E. R., Hiemstra, J. F., and Auton, C. A.: Subglacial till: Formation, sedimentary characteristics and classification, Earth-Sci. Rev., 78, 115–176, 2006.
Gehrmann, A.: The multi-stage structural development of the Upper Weichselian Jasmund glaciotectonic complex (Rügen, NE Germany), Doctoral thesis, University of Greifswald, 1–235, 2018.
Gehrmann, A. and Harding, C.: Geomorphological mapping and spatial analyses of an Upper Weichselian glacitectonic complex based on LiDAR data, Jasmund Peninsula (NE Rügen), Germany, Geosciences 8, 208, https://doi.org/10.3390/geosciences8060208, 2018.
Gehrmann, A., Meschede, M., Hüneke, H., and Pedersen, S. A. S.: Sea cliff at Kieler Ufer (Pleistocene stripes 11-16) – Large-scale architecture and kinematics of the Jasmund Glacitectonic Complex, DEUQUA Spec. Pub., this volume, 2019.
Hiemstra, J. F. and Rijsdijk, K. F.: Observing artificially induced strain: implications for subglacial deformation, J. Quarternary Sci., 18, 373–383, 2003.
Kanter, L.: Der M2-Till von Nordost-Rügen, Diploma-Thesis, University of Greifswald, Inst. of Geogr. and Geol., 1–62, Greifswald, 1989.
Kenzler, M. and Hüneke, H.: Sea cliff at Glowe: Stratigraphy and absolute age chronology of the Jasmund Pleistocene sedimentary record, DEUQUA Spec. Pub., this volume, 2019.
Kenzler, M., Tsukamoto, S., Meng, S., Frechen, M., and Hüneke, H.: New age constraints from the SW Baltic Sea area – implications for Scandinavian Ice Sheet dynamics and palaeo-environmental conditions during MIS 3 and early MIS 2, Boreas, 46, 34–52, 2017.
Kielczynski, S.: Interpretation of bed forms from sedimentary structures: lateral and vertical facies relationships of the I2 sand deposits at Dwasieden (Island of Rügen), Master thesis, Universität Greifswald, 1–129, Greifswald, 2016.
Krienke, K.: Das Geschiebeinventar der weichselhochglazialen Tills von Südostrügen- Hilfsmittel zur Lithostratigraphie sowie zur Rekonstruktion von Ablagerungsbedingungen und glazialer Dynamik, Archiv für Geschiebekunde, 3, 701–710, 2004.
Lachniet, M. S., Larson, G. J., Lawson, D. E., Evenson, E. B., and Alley, R. B.: Microstructures of sediment flow deposits and subglacial sediments: a comparison, Boreas, 30, 254–262, 2001.
Larsen, E., Piotrowski, J. A., and Menzies, J.: Microstructural evidence of low-strain, time-transgressive subglacial deformation, J. Quarternary Sci., 22, 593–608, 2006.
Ludwig, A. O.: Eistektonik und echte Tektonik in Ost-Rügen (Jasmund), Wissenschaftliche Zeitschrift Universität Greifswald, 4, 251–288, 1954/1955.
Ludwig, A. O. and Panzig, W.-A.: Stopp 5: Das Pleistozän südlich Sassnitz – Fazies und Lagerung glazilimnischer/-fluviatiler Sedimente am Kliff bei Dwasieden, in: Eiszeitlandschaften in Mecklenburg-Vorpommern, edited by: Lampe, R. and Lorenz, S., 68–69, Geozon, Greifswald, 2010.
Murton, J. B.: Near-Surface Brecciation of Chalk, Isle of Thanet, South-East England a Comparison with Ice-Rich Brecciated Bedrocks in Canada and Spitsbergen, Permafrost Periglac., 7, 153–164, 1996.
Panzig, W.-A.: Das geschiebeinhaltliche Normalprofil des Till-Inventars von NE-Rügen und stratigraphische Konsequenzen im Ergebnis des Versuchs einer Tilldecken-Regionalkorrelation im SW-lichen Ostseegebiet auf geschiebekundlicher Grundlage, Habilitation-Thesis, University of Greifswald, Inst. of Geogr. and Geol., 1–149, Greifswald, 1989.
Phillips, E., van der Meer, J. J. M., and Ferguson, A.: A new “microstructural mapping” methodology for the identification, analysis and interpretation of polyphase deformation within subglacial sediments, Quaternary Sci. Rev., 30, 2570–2596, 2011.
Phillips, E., Spagnolo, M., Pilmer, A. C. J., Rea B. R., Piotrowski, J. A., Ely, J. C., and Carr, S.: Progressive ductile shearing during till accretion within the deforming bed of a palaeo-ice stream, Quaternary Sci. Rev., 193, 1–23, 2018.
Pisarska-Jamroży, M., Belzyt, S., Börner, A., Hoffmann, G., Hüneke, H., Kenzler, M., Obst, K., Rother, H., and van Loon, A. J.: Evidence from seismites for glacio-isostatically induced crustal faulting in front of an advancing land-ice mass (Rügen Island, SW Baltic Sea), Tectonophysics, 745, 338–348, 2018a.
Pisarska-Jamroży, M., van Loon, A. J., and Bronikowska, M.: Dumpstones as records of overturning ice rafts in a Weichselian proglacial lake (Rügen Island, NE Germany), Geol. Q., 62, 917–924, 2018b.
Strahl, U.: Über die “Ablationsmoräne” des M1- Geschiebemergels Jasmunds und Arkonas (Rügen), Diploma thesis, University of Greifswald, Sektion Geologische Wissenschaften, 1–65, Greifswald, 1988.
van der Meer, J. J. M.: Microscopic Evidence of Subglacial Deformation, Quarternary Sci. Rev., 12, 553–587, 1993.
Waller, R., Phillips, E., Murton, J., Lee, J., and Whiteman, C.: Sand intraclasts as evidence of subglacial deformation of Middle Pleistocene permafrost, North Norfolk, UK, Quaternary Sci. Rev., 30, 3481–3500, 2011.