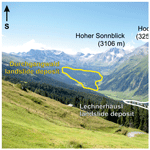
Glaciers and mass movements in the Hüttwinkl Valley (Hohe Tauern range): from the Last Glacial Maximum (LGM) until now
Jürgen M. Reitner
Mathias Steinbichler
Reitner, J. M. and Steinbichler, M.: Glaciers and mass movements in the Hüttwinkl Valley (Hohe Tauern range): from the Last Glacial Maximum (LGM) until now, DEUQUA Spec. Pub., 5, 13–30, https://doi.org/10.5194/deuquasp-5-13-2024, 2024.
The Hüttwinkl Valley, the uppermost section of the Rauris Valley, offers a unique sedimentary and morphological archive to study the development of the landscape in a high-Alpine valley since the Last Glacial Maximum (LGM). Glacial extents of the past, especially during the Younger Dryas (12.8–11.7 ka) and the Little Ice Age, as well as the present-day massive glacier retreat, are clearly recorded. On the other hand, the effects of different mass movements on valley development are evident. The field trip begins in Kolm-Saigurn, where the Durchgangwald landslide occurred in the Bølling–Allerød interstadial (14.7–12.9 ka). In the Younger Dryas (12.9–11.7 ka) glaciers overflowed parts of the Durchgangwald landslide deposit followed by the Lenzanger landslide (Preboreal, Early Holocene). North of Kolm-Saigurn, the Bucheben landslide (Early Holocene) and the Lechnerhäusel landslide (Middle Holocene) showed first a rockslide phase followed by a rock avalanche (sturzstrom), which led to the formation of toma in the distal area. All landslides detached from the western flank of the valley, whereas the eastern slopes are predominantly characterized by slow, deep-seated gravitational slope deformation (DSGSD). The most recent significant mass wasting was caused by rockfalls and catastrophic debris flows.
- Article
(21282 KB) - Full-text XML
- BibTeX
- EndNote
The Hüttwinkl Valley, the upper part of the Rauris Valley, drained by the creek Hüttwinkl Ache, is one of the most outstanding valleys of the Eastern Alps regarding the impact of different types of mass movements on landscape development. In addition, moraines and glacially shaped landforms in the forefield of rapidly decaying glaciers result in a picturesque scenery. The aim of the field trip is to show this unique sedimentary and morphological archive and demonstrate how the chronology of the contributing glacial, gravitational and fluvial processes have been deciphered so far. We present partly preliminary research performed in the course of the geological survey for the geological map sheet ÖK 154 Rauris.
This contribution provides a short overview of the morphological and geological setting (Sect. 2; Figs. 1, 2, 3), followed by a brief summary of the landscape development known so far with a special focus on mass movements and valley floor development (Sect. 3). A more detailed description of the sites will be given in the following chapters in order of their appearance during the field trip. It starts with the first location at Kolm-Saigurn (A in Figs. 1, 2; Sect. 4; Figs. 4, 5, 6, 7, 8) followed by the description of the landslides north of Bodenhaus (Sect. 5), including the Lechnerhäusl landslide (B in Figs. 1, 2; Figs. 9, 10) and the Bucheben landslide (Figs. 11, 12). Finally, the various cases of deep-seated gravitational slope deformation (DSGSD; term used in the sense of Dramis and Sorriso-Valvo, 1994, and Agliardi et al., 2001) on the eastern valley flank, which are evident throughout the field trip, will be discussed in Sect. 6 (Fig. 12).
Last Glacial Maximum (LGM) is used in the sense of the Würmian Pleniglacial and covers the time span of 29 to ca. 19 ka (see Reitner, 2022; van Husen and Reitner, 2022, with further references). The subdivision of the Würmian or Alpine Lateglacial (ca. 19–11.7 ka) into glacial stadial follows the suggestion by Reitner et al. (2016). Holocene stages and subepoches are classified according to Walker et al. (2018).
2.1 Topographical conditions and highlights
The catchment of the Hüttwinkl Valley (valley of the Hüttwinkl Ache creek) reaches from the highest and most prominent peaks (Hocharn 3254 m, Hoher Sonnblick 3106 m) to the confluence with the Seidlwinkl Ache at the village of Wörth (part of the community of Rauris) and thus to the onset of the Rauriser Ache creek (Figs. 1, 2, 3). The uppermost cirques of the catchment are still glaciated with the most prominent glaciers (called “Kees” in the local dialect) of Goldbergkees and Pilatuskees below the Hoher Sonnblick peak and Hocharnkees and Krumlkees below the Hocharn peak. The glaciological monitoring of the Goldbergkees started already in the year 1896 (Penck, 1897), which allowed us to document the effect of climate change on the glacier size in great detail since then (Böhm et al., 2007; Auer et al., 2010; Hansche et al., 2023)
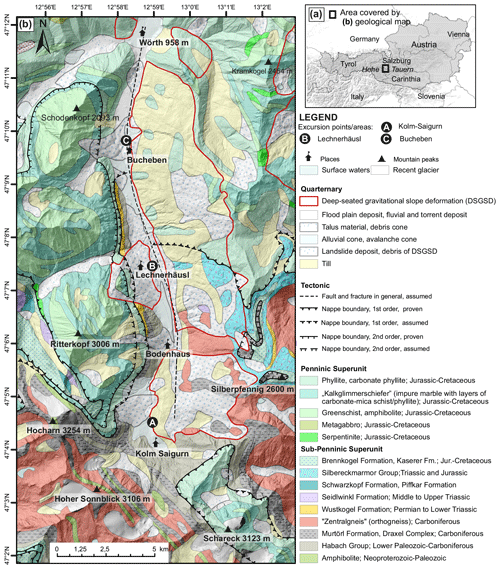
Figure 1(a) Location of the Hüttwinkl Valley in the Province of Salzburg, in the Hohe Tauern mountain group (Eastern Alps) is marked. (b) Geological overview based on Pestal et al. (2005) with the main sites of the field trip (A – Kolm-Saigurn; B – Lechnerhäusl landslide; C – Bucheben landslide) indicated. Sources: Hillshade – Geoland Basemap (http://geoland.at, last access: 10 June 2024); Geology: Geologische Bundesanstalt Österreich (2022).
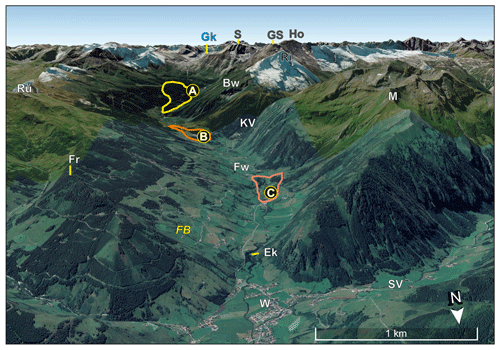
Figure 2View of the Hüttwinkl Valley towards the south showing the valley asymmetry with steep west-facing slopes and more gentle slope on the east-facing side. Important sites are indicated. Field trip locations are plotted on a Google Earth image (with black circle, © Google Earth 2024); A – Kolm-Saigurn; B – Lechnerhäusl landslide; C – Bucheben landslide. Further locations: Bw – Bocksteinwand rock wall; Ek – Einödkapelle (1012 m); FB – Fröstlberg slope; Fr – Fröstlberg (1823 m); Fw – Frohnwirt (1074 m); Grieswies-Schwarzkogel (3116 m); Ho – Hocharn (3254 m); KV – Kruml Valley; M – Mitterkarkopf (2404 m); Ri – Ritterkopf (3006 m); Rü – Rührkübel (2482 m); S – Hoher Sonnblick (3106 m); SV – Seidlwinkl Valley.
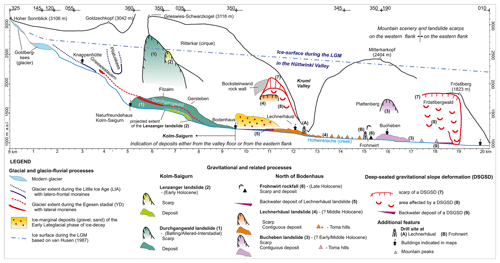
Figure 3Section along the Hüttwinkl Valley from the Hoher Sonnblick peak to the confluence with the Seidlwinkl Ache creek showing main elements for understanding the landscape evolution.
Such an early scientific investigation was enabled by the last attempts of gold mining lasting some centuries (Gruber, 2004). In 1886, Ignaz Rojacher, the last gold mining entrepreneur and open-minded adventurer, constructed the, by that time, highest meteorological observatory on the summit of the Hoher Sonnblick (Böhm et al., 2011). This Sonnblick Observatory, now run by GeoSphere Austria, is an outstanding research infrastructure for studying high-atmospheric processes and climate change.
2.2 Geological framework
The Hüttwinkl Valley is located in the eastern part of the so-called “Tauern Window”. The Tauern Window exposes the lower-plate Penninic and Sub-Penninic superunits that were buried during the Alpine subduction and collision below the upper-plate Austroalpine Superunit (Schmid et al., 2004). With its size of 160 × 30 km, it is the biggest of the intra-Alpine tectonic windows. In addition to preserved thrust contacts, the limits of the Tauern Window are to the west and east the Brenner and Katschberg normal faults, to the north the Salzachtal–Ennstal fault zone, and to the southeast the Mölltal fault zone. The northern part of the Hüttwinkl Valley is mainly built up by Penninic rocks and consists of Jurassic to Cretaceous oceanic meta-sediments as well as mafic and ultramafic rocks deriving from an oceanic lithosphere. The southern part of the Hüttwinkl Valley on the other hand consists of rocks of the Sub-Penninic Superunit and is built up by pre-Variscan crystalline complexes, Carboniferous orthogneiss and Carboniferous to Cretaceous meta-sediment formations (Pestal et al., 2005; Schuster et al., 2014). The lithological information is used to understand prominent landscape-forming events like the Durchgangwald landslide, as, e.g., the lithological succession of the scarp area of the landslide is preserved in the landslide deposits. Furthermore, the main glaciers of the Hüttwinkl Valley (Goldbergkees and Pilatuskees) are located more or less in areas where the border between the Penninic to Sub-Penninic superunits crops out. Hence, the finding of sub-rounded to rounded “Zentralgneis” (carboniferous orthogneiss; see Fig. 1) components in glacial sediments downstream of the bedrock is indicative of transport during Lateglacial and Holocene advances and retreats (Bichler et al., 2016; Pestal et al., 2005).
Descriptions and explanations of the landscape of the Hüttwinkl Valley in the context of bedrock geology, glacial shaping and mass movements have been provided by Hottinger (1935), Exner (1957) and von Poschinger (1986). Especially the pronounced valley asymmetry (see Fig. 2) consisting of a more gentle slope on the eastern flank (average 20°; according to Fellner, 1993) and a steeper western flank (average 35°; based on Fellner, 1993) leads to different explanations. Hottinger (1935), who for the first time discovered the large landslide deposits of Kolm-Saigurn, Lechnerhäusl and Bucheben descending from the steep western flank, explained the overall morphology with the general trend of the main planar fabric (foliation, schistosity): the gentle slopes on the true right flank follow the dip towards west and show slow mass movements. In contrast, the steep true left flank where the scarp of the landslides is located is made up of basset edges. Exner (1957) mapped large areas affected by mass movements on the eastern flank from Fröstlberg southward to east of Bodenhaus, which can be classified in modern terminology as DSGSDs. However, he regarded most DSGSDs to be of interglacial (= pre-LGM) age, as large areas are covered by an LGM till some meters thick (see Figs. 9, 11). Already von Poschinger (1986) showed that Hottinger's (1935) explanation of the morphological asymmetry appears to be oversimplified, as the main planar fabric (foliation, schistosity) shows for large areas (approx. from the Kruml Valley and Lechnerhäusl northward) a gentle northward dip. His results as well as those of Fellner (1993) show the importance of the joint orientation together with that of the main planar fabric (foliation, schistosity) as a relevant cause for the formation of DSGSDs and hence the valley morphology.
Based on the existing reconstruction of the LGM ice extent (van Husen, 1987), the Hüttwinkl Valley was part of the large network of valley glaciers with an ice surface along the valley axes between 2800 and 2300 m a.s.l. (see Fig. 3). However, well-developed trimlines of the LGM have not been found in the Hüttwinkl Valley due to comparable weak lithologies like different types of schists, which are not able to preserve the record of glacial erosion (compare with Penck and Brückner, 1909). In addition, different types of mass movements altered the subglacial landscape shaped during the LGM. In total, glacial shaping had a limited influence on the valley morphology as indicated by the valley asymmetry (von Poschinger, 1986). The most prominent legacy of the LGM exists with an extensive cover by subglacial tills on the eastern flank (compare with Exner, 1957; Figs. 9, 11).
Delta foreset beds consisting of sandy to gravelly clinoforms and silty glaciolacustrine deposits are present in some areas from the valley floor up to ca. 1600 m a.s.l. (Figs. 3, 9, 10e). Such deposits were formed in ephemeral ice-dammed lakes at the margin of rapidly downmelting glaciers during the phase of ice decay in the early Lateglacial (Reitner, 2007; Reitner et al., 2016), when the LGM ice masses of the Eastern Alps collapsed between 19 and 18 ka (Klasen et al., 2007; Wirsig et al., 2016).
Latero-frontal moraines of the following Gschnitz stadial (17–16 ka; Ivy-Ochs et al., 2023a, with references), which represents the last glacier advance before the Bølling–Allerød Interstadial (14.7–12.9 ka), are missing in the Hüttwinkl Valley in contrast to quite frequent documented instances in the Hohe Tauern mountain range elsewhere (see van Husen and Reitner, 2022). Direct removal or coverage by catastrophic landslides and the infill of landslide-dammed lakes resulting in drowned landscapes most likely erased all landforms of the Gschnitz paleoglaciers.
No time markers for the (re-)activation of the DSGSDs after the LGM have been identified so far (von Poschinger, 1986). Evidence from Kolm-Saigurn (see below) indicates an onset of such DSGSDs before the Bølling–Allerød Interstadial (14.7–12.9 ka).
According to 10Be surface exposure dating, the Durchgangwald landslide (Bichler et al., 2016; Figs. 3, 4, 5, 6, 7, 8) happened around 13 ka at the end of the Bølling–Allerød Interstadial (14.7–12.9 ka). Von Poschinger (1986) could show that the emplacement of the 400 ×106 m3 large landslide mass (Bichler et al., 2016) at the toe of the eastern slope most likely stopped the further development of a previously formed DSGSD (at Durchgangriegel; Fig. 12b).
During the Younger Dryas (YD; 12.9–11.7 ka), the last climatic deterioration of the Würmian Lateglacial, the cirque glaciers showed the prominent re-advance of the Egesen stadial. The precursors of Goldberger and Pilatuskees merged together at Kolm-Saigurn and overflowed parts of the Durchgangwald landslide deposit. A series of recessional moraines show the further development during the Egesen stadial before the onset of the Holocene. Latero-frontal moraines of the Egesen stadial are quite frequent in the Hüttwinkl catchment and show in many cases the last glacier occupation of present-day non-glaciated cirques. In the older literature (e.g., Exner, 1957), such moraines are attributed to the Daun stadial, which is now included in the term Egesen stadial (see Reitner et al., 2016).
At Kolm-Saigurn, the Early Holocene Lenzanger landslide (10Be age of ca. 11 ka; Preboreal; Bichler et al., 2016; Figs. 3, 4, 6) covered the subglacial till of the Egesen stadial, which finally resulted in a unique sequence of superpositions where a YD till is part of a sequence from the Bølling–Allerød Interstadial to the early Holocene (compare also with Ivy-Ochs et al., 2023b).
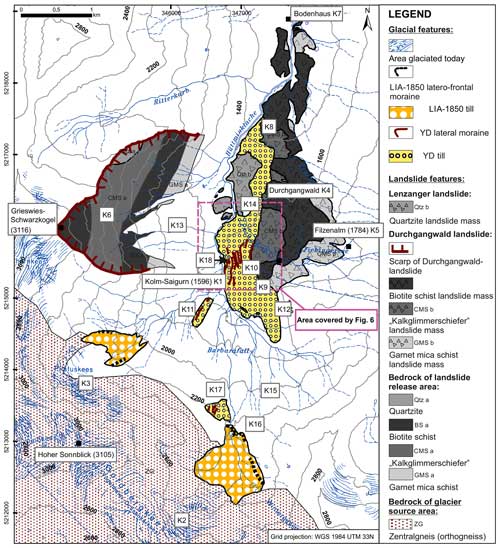
Figure 4Simplified topographical and geological overview of the study area, showing the most important features of the study area. Topographical features referred in this study are marked on the map as (K). Numbers given on contour lines and next to topographical names indicate elevation in meters above sea level (m a.s.l.; modified after Bichler et al., 2016). The extent of Fig. 6 is indicated.
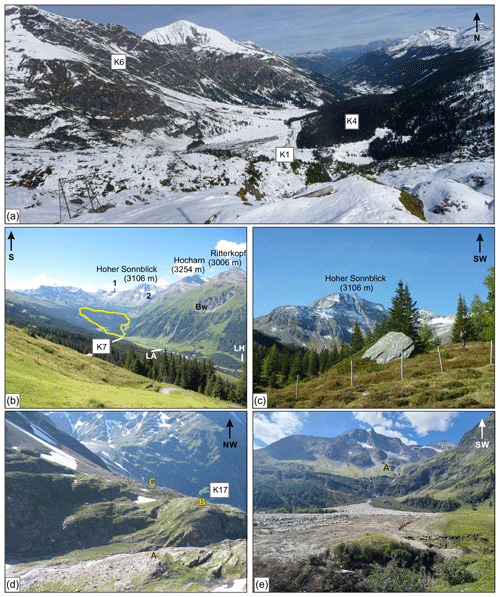
Figure 5Photographs of the Kolm-Saigurn area ((a), (c), (d) are modified after Bichler et al., 2016). Locations are marked according to Fig. 4. (a) View from K17 towards the north. The scarp area of the Durchgangwald landslide (K6) and its main mass (K4) onlapping on the opposite valley side is visible. (b) View towards the south of the whole study area. Note the still glaciated areas of the Goldbergkees (1) and Pilatuskees (2) in the background. The extent of the landslide area is highlighted in yellow. LH – location of Lechnerhäusl inn; LA – quarry north of Lohningeralm (see Fig. 9). (c) Most distal part of the Durchgangwald landslide, at the Filzenalm (K5). The boulder in the photograph is a 10Be-dated boulder from Bichler et al. (2016). (d) View at the forefield of the LIA-1850 terminal moraine of the Goldbergkees. A is terminal moraine; B is plateau of K17 with several dated boulders and dated quartz veins in polished bedrock; C is four lateral moraine ridges at K17 belonging to the Kolm-Saigurn glacier system of Younger Dryas (YD) age. (e) View at the LIA moraine (A) of Pilatuskees, which was partly mobilized in the course of the heavy rain event on 28 August 2023. Debris flow deposits in the foreground (image by Marc Ostermann).
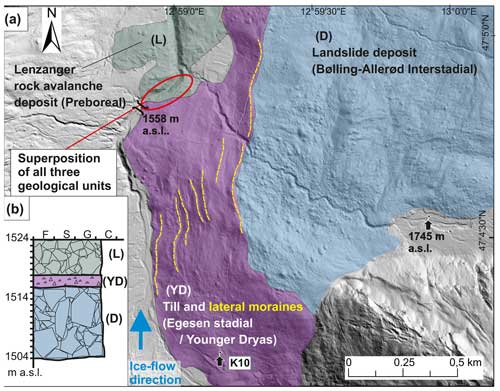
Figure 6(a) Hillshade image showing the morphological contrast between the rugged terrain of the interstadial Durchgangwald rockslide deposit (D) and the subglacially smoothed landscape with multiple latero-frontal moraines formed during the Egesen stadial (YD; location indicated by Fig. 4). The youngest rock avalanche event (Lenzanger (L)) is evident in the northwest. K10 – Ammererhof. (DEM provided by the government of Salzburg Province.) (b) The site with the superposition of all three units (inset stratigraphic profile) is indicated (modified after Ivy-Oches et al., 2023b, and Bichler et al., 2016).
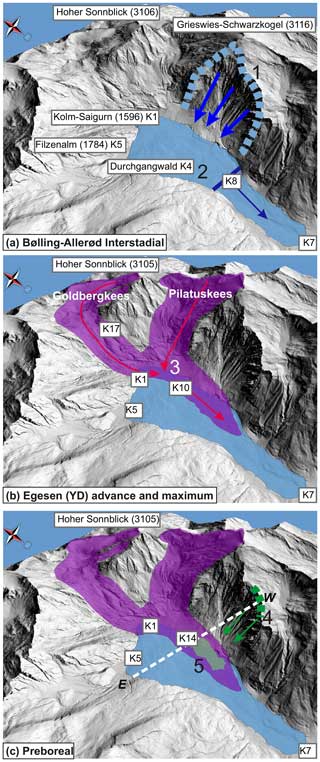
Figure 7Visualization of the extent, origin and movement direction of the Durchgangwald landslide (a), the Kolm-Saigurn glacier system (b) and the Lenzanger landslide (c). (a) 1 is the scarp of the Durchgangwald landslide; 2 is the border at the Gersteben step (K8) where the kinematics of the landslide changed from sliding to dynamic flow in the sense of a rock-avalanche and the flow direction changed from east to north. (b) 3 is the confluence of the Goldbergkees and Pilatuskees in Kolm-Saigurn. (c) 4 is the supposed scarp area of Lenzanger landslide; 5 is the Lenzanger landslide mass. The dashed line marks the approximate location of the cross-section in Fig. 8. Locations (K) as indicated in Fig. 4: K1 – Kolm-Saigurn Naturfreundehaus; K5 – Filzenalm; K7 – Bodenhaus; K10 – Ammererhof; K14 – Lenzanger; K17 – plateau in the forefield of Goldbergkees. (Figure modified after Bichler et al., 2016; DEM provided by the government of Salzburg Province.)
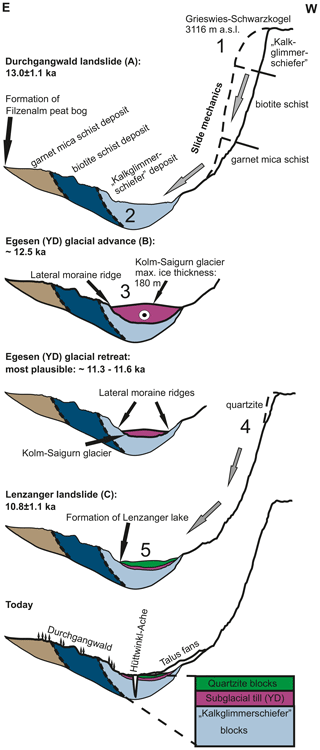
Figure 8Generalized landscape evolution sketch, indicated in Fig. 7. Numbers and letters are according to Fig. 7. Features are not to scale. (Figure modified after Bichler et al., 2016.)
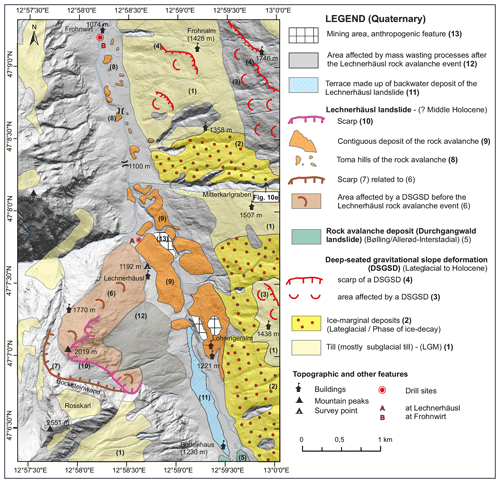
Figure 9Simplified geological map of the Lechnerhäusl landslide and surrounding. The extent of the till cover is partly based on Griesmeier (2021). Source: Hillshade – Geoland Basemap (http://geoland.at).
Down-valley of Bodenhaus, where the most distal parts of the Durchgangwald landslide are evident (Figs. 4, 9), the chronology of mass movements (landslides and DSGSDs) can be constrained only relatively.
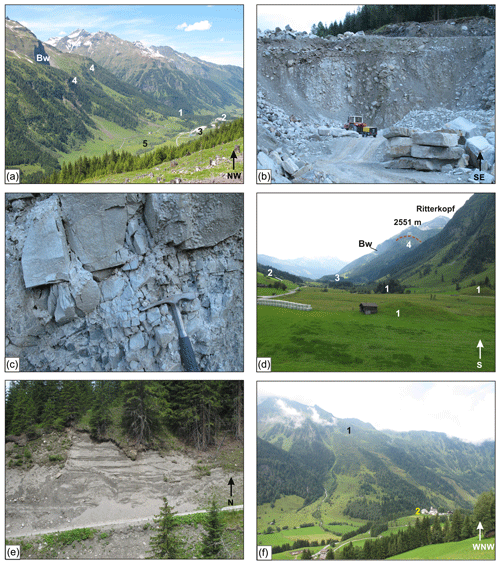
Figure 10(a) View at the Lechnerhäusl landslide from the eastern valley flank. The landslide deposit at Lechnerhäusl (1) and the quarries in the landslide deposit (2 – northeast of Lechnerhäusl; 3 – north of Lohningeralm) are evident. The run of the supposed scarp (4) below the Bocksteinwand (Bw) rock wall) is less clear in contrast to the backwater terrace of the Lechnerhäusl landslide (5). (b) Landslide deposit at the quarry northeast of Lechnerhäusl (no. 2 in (a)). Vertically dipping of strongly fragmented phengite gneiss. (c) Detail of a strongly fragmented phengite gneiss showing fractures which were caused by dynamic fragmentation resulting in the formation of jigsaw-clasts. (d) View to the south with small hills, so-called toma (1), made up of Lechnerhäusl rock avalanche deposits. Their position is mostly on the valley floor. In one case (2) such a deposit was deposited at the toe of a slope. (3) – Quarry northeast of Lechnerhäusl. (4) – scarp of the DSGSD which was formed before the Lechnerhäusl-landslide. Bw – Bocksteinwand rock wall. (e) Delta foresets structures indicating synsedimentary deformation typical of ice-marginal deposits of the early Lateglacial phase of ice decay (Mitterkarlgraben in 1575 m a.s.l., indicated in Fig. 9). (f) View at the scarp (1) and landslide deposit (2) of Bucheben (with chapel of Bucheben).
The Bucheben landslide (Figs. 3, 11) occurred most likely at the end of the Early Holocene (Greenlandian; 11.7–8.3 ka), based on the 14C-dated drilling results in the backwater area (drill site B). As the toma hills of the Bucheben landslides lay on top of the backwater terrace level of the Fröstlberg DSGSD, the latter must be older.
The Lechnerhäusl landslide (Figs. 3, 9) happened after the Bucheben landslide as its toma hills were deposited on the valley floor level formed in the backwater area of the Bucheben landslide. A dated base of a peat drilled at a mire within the landslide area at Lechnerhäusl (drill site A) indicates a landslide event during the Middle Holocene (Northgrippian; 8.3–4.2 ka). The damming of the Hüttwinkl creek resulted as well in the formation and infill of a lake, which most likely drowned the most distal deposits of the Durchgangwald landslide south of Bodenhaus (Bichler et al., 2016).
Smaller rockfall events happened of course afterwards but had no impact on the development of the valley floor. The only exception is the small rockfall deposit at Frohnwirt (Fig. 3, 11), which may have led to a short-lasting disturbance of the river gradient in the Late Holocene (Meghalayan; 4.2–0 ka).
Catastrophic debris flows of mostly limited extent occur frequently. The disintegrated rock of the DSGSD slopes (Fig. 13c), as well as tills, provides sufficient debris for such catastrophic events (Baur et al., 1992), as demonstrated by the last event on 28 August 2023 (Fig. 5e).
At Kolm-Saigurn (Figs. 1, 3, 4, 5, 6) three well-distinguishable geological and geomorphological units allow a reconstruction of gravitational and glacial processes and the establishment of chronology (Bichler et al., 2016). These units are, in chronological order,
-
the Durchgangwald landslide
-
the tills and moraines of the Kolm-Saigurn glacier system
-
the Lenzanger landslide.
During the field trip, the morphological and sedimentary expression of all three units will be presented.
4.1 The Durchgangwald landslide
The 10Be surface exposure dating and geological reconstructions (Bichler et al., 2016) showed that the Durchgang landslide occurred at 13.0 ± 1.1 ka in an ice-free environment at the end of the Bølling–Allerød Interstadial, just before the onset of the Younger Dryas. The 0.4 km3 landslide mass (Figs. 4, 5, 7, 8) detached from the glacially oversteepened eastern flank of the Grieswies-Schwarzkogel (3116 m) and covers an area of ca. 3.9 km2. By comparing the lithological succession at the flank consisting of westward-dipping garnet mica schist, “Kalkglimmerschiefer” (meaning impure marble with layers of carbonate–mica schist and phyllite) and biotite mica schist, with the similar but rotated succession of the landslide deposit east of the Hüttwinkl creek, the detachment and movement occurred along one distinct displacement plane as proposed by Hottinger (1935) and von Poschinger (1986). The upper part of the plane has an inclination of 40–50° and is thus less steep than the 70–80° joints in the scarp area (Fig. 8), indicating a new formation of the plane (von Poschinger, 1986). Outcrops of the landslide deposit and the geological evidence of the water tunnel running through the mass (see also Dirnberger and Hilberg, 2020; Fig. 12) document a slightly rotated, i.e., steeper, dip with more or less the same dip direction, indicating a rotational component of the movement. The rugged surface of the landslide (Fig. 6) consists of clast-supported boulder-sized scree made up of angular to subangular, sometimes house-sized Kalkglimmerschiefer blocks. The forested area is also characterized by a large number of small standing water and waterlogged areas (see also hydrogeological investigations by Dirnberger and Hilberg, 2020), which leads to the local name “Rauriser Urwald” (primeval forest of Rauris or “Rauris virgin forest”).
The northern sector of the landslide comprises compressional structures, as evident in the field and on the DEM. These morphological features indicate a fast, dynamic flow in the sense of a rock avalanche (sturzstrom) for the most distal and down-valley part of the landslide mass, towards the Bodenhaus (K7) location. Such a change in the landslide kinematics might have been enabled by the successive increase in fragmentation with transport distance towards the distal part and by a supposed pre-existing step in the bedrock in the valley (at K8), leading to a steeper gradient in this area. The calculated Fahrböschung (meaning travel angle; Heim, 1932) for the main sliding part is an 11 to 12° angle with a run-out length of ∼ 4.1 km. Including the rock avalanche leads to a run-out length of around 5.6 km and a travel angle of ∼ 14°. However, the latter number has to be considered an upper limit, as the most distal part of the rock avalanche is covered by backwater sediments derived from the Lechnerhäusl landslide (Sect. 5.1; Figs. 3, 9). Altogether, the Durchgangwald landslide resulted in the blocking of the Hüttwinklache creek in the area of Kolm-Saigurn (K1) and of the creeks at the Filzenalm (K5), with lakes and bogs subsequently forming at its margin (Fig. 7).
4.2 The Kolm-Saigurn glacier system
At the onset of the Younger Dryas (YD), glaciers re-advanced into the valley head of both the Pilatuskees (K3) and Goldbergkees (K2) (Fig. 7). During this prominent glacier advance the western part of the Durchgangwald landslide got overridden, as proven by a cover of consolidated, massive and matrix-supported diamict with occasional striated clasts, a subglacial till typical of a temperate glacier (Fig. 4). Zentralgneis and Kalkglimmerschiefer dominate the clast lithology. On the slope north of the Ammererhof (K10), a series of nine parallel latero-frontal moraines, mostly with Zentralgneis boulders on top, can be mapped, with the highest marking the border to the un-topped Durchgangwald landslide deposits (Fig. 6). Based on the morphological and sedimentary features, the maximum extent of the YD advance and the recessional phase with glacier stillstands and stabilization can be reconstructed. However, no end moraine exists anymore at the maximum position south of Bodenhaus, most likely due to fluvial action, which eroded also big parts of the glacial evidence on the true left flank.
The most remarkable succession of four lateral moraines of YD age occurs on a plateau at 2250–2200 m (K17) in the forefield of the Little Ice Age (LIA) – moraines of the Goldbergkees (K16) and thus high above the field trip route (Fig. 4d). Their position and altitude allow an approximation on the paleo-equilibrium-line altitude (ELA) during the YD maximum based on the maximum elevation of lateral moraine approach (Lichtenecker, 1938).
The age model of the YD Kolm-Saigurn glacier system is based on the superposition of the 10Be-dated Durchgangwald landslide and the 10Be exposure dates of eight boulder samples and two bedrock samples (for details see Bichler et al., 2016). The 14C-dated base of a peat formed after the glacier advance of Melcherböden with an age of 11.35–11.20 ka cal BP provides the upper age constraint of the YD glacier advance.
4.3 The Lenzanger landslide
Around the Grieswiesalm (K13) and Lenzanger (K14) area, the till cover is topped by a clast-supported scree with angular quartzite boulders reaching a size of up to 10 m diameter (Figs. 4, 6; Bichler et al., 2016). The surface morphology is characterized by a series of curved ridges, indicating a flow-like movement. The quartzite blocks cover an overall surface area of around 0.3 km2. The source of these blocks has been interpreted to document a landslide or, more precisely, a rock avalanche, originating from the western valley flank, similar to the Durchgangwald landslide but much smaller in scale. The stratigraphical succession (Figs. 1, 3, 6) and the preservation of typical rock avalanche features indicate that this rapid mass movement happened in an ice-free environment after the YD glacier advance and retreat. This conclusion is supported by the 10Be ages of two boulders giving a mean age of 10.8 ± 1.1 ka which falls into the Preboreal (Early Holocene).
4.4 Debris flow event of 28 August 2023
In the course of the field trip, we will see the legacy of a very recent debris flow event which devastated the valley floor of Kolm-Saigurn (Fig. 5e). On 28 August 2023, a massive debris flow mobilized some 100 000 m3 of Pilatuskees LIA moraine triggered by a heavy rain event (unpublished data provided by Melina Frießenbichler, Daniel Binder, Bernhard Hynek, Anton Neureiter, Georg Pistotnik, Marc Ostermann, Gernot Weyss). The debris flow is part of a process cascade that has even reached Rauris about 16 km downstream.
5.1 Lechnerhäusl landslide
The investigation of the Lechnerhäusl landslide event has not been performed in such detail as that of Kolm-Saigurn. Hottinger (1935) provided a brief description of the scarp and the extent of the deposits. Exner (1957) assumed a Gschnitz moraine within the area of the landslide, whereas von Poschinger (1986) described the lithology of the deposits. Abele (1974) named the landslide Bocksteinwand–Lechnerhäusl as he, like Exner (1957), regarded the rock wall of Bocksteinwand as the head scarp. However, such a release area seems to be unlikely according to the map of Hellerschmidt-Alber (1998). Taking into account his results and the modern DEM, the assumed extent of the scarp is displayed in Fig. 9. The lithological units in the surrounding stable areas show a shallow to moderate dip from the southwest to northwest.
An extensive scarp below the Bocksteinwand represents the release area of a large DSGSD which resulted in a downward movement of a rock mass consisting of Kalkglimmerschiefer, prasinite, dark phyllite and so-called “phengite gneiss” of the Wustkogel Formation. Eventually the scarp of the Lechnerhäusl landslide developed on the southern part of the rock mass affected by the DSGSD (Figs. 9, 10a).
More or less contiguous bodies of landslide deposits can be mapped east of Lechnerhäusl and on the opposite (eastern) valley flank from Lohningeralm northward. In general, a lithological succession from west to east with Kalkglimmerschiefer, dark phyllites and quartzite, phengite gneiss, and prasinite with dark phyllite occurs within the landslide debris (von Poschinger, 1986). Large angular boulders of Kalkglimmerschiefer are characteristic east of Lechnerhäusl. Quarries for natural stone slabs northeast of Lechnerhäusl and north of Lohningeralm expose phengite gneiss. The original set of discontinuities (joints, foliation, schistosity, faults) is still discernible, but the dip shows a strong variation, and features typical of dynamic fragmentation (in the sense of McSaveney and Davies, 2006) like jigsaw clasts (see Dufresne et al., 2016) are present (Fig. 10b, c).
Isolated hills, so-called toma, occasionally sitting on the toe of the eastern flank but mostly on top of the flat valley floor represent the distal part of the landslide (Figs. 9, 10d). Those toma with a height of a few meters consist mostly of Kalkglimmerschiefer but also of prasinite, dark phyllite and phengite gneiss (Wustkogel Formation).
A preliminary kinematic analysis based on field evidence indicates that an initial rockslide with a newly formed plane hit the opposite valley flank. Increasing dynamic fragmentation soon led to the transformation into a typical rock avalanche as indicated by the depositional features and the toma hills. The latter “surfed” on top of the valley floor which was formed in the backwater of the Bucheben landslide.
No calculation of the volume detached during the landslide has been performed so far. Abele (1974) estimated the area at 3 km2 and calculated the travel length (5.5 km) and the Fahrböschung (12.5°) based on a proposed scarp detachment from the Bocksteinwand. We reconstructed a contiguous landslide area of 1.5 km2, which did not include the areas below the scarp which were affected by slope processes after the landslide event. Depending on the assumed highest scarp, the travel length varies between 4.7–5.2 km, resulting in a Fahrböschung from 8.4 to 10.2°.
According to the situation along the Hüttwinkl valley, the Lechnerhäusl landslide is younger than the landslides of Durchgangwald and Bucheben. A drill core at the mire northeast of Lechnerhäusl (drill site A; Figs. 3, 9) consisted of sandy material ca. 4.7 m below the surface containing a small amount of mostly undefinable plant material. The 14C dating provided an age of ca. 5.3–5.0 ka cal BP indicating that the Lechnerhäusl landslide most likely occurred in the Middle Holocene (8.3–4.2 ka). Such a quite young age fits well to the morphological evidence, as the perturbation of the river gradient of the Hüttwinkl creek in this flat area is still prominent (Fig. 3).
In the course of the field trip, we will make a short walk to the former upper mining area east of Lohningeralm where we see landslide deposits and adjoining ice-marginal deposits. From this point, we get an excellent overview of the Lechnerhäusl scarp area, the backwater area and the distal part of Durchgangwald landslide.
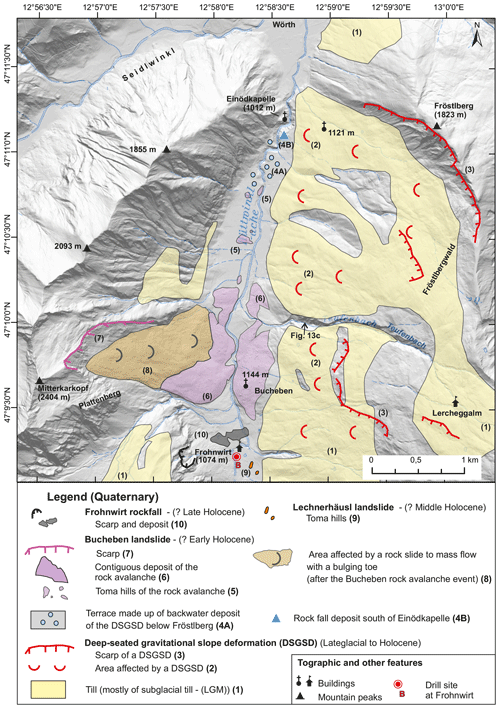
Figure 11Simplified geological map of the Bucheben landslide and surrounding. The extent of the till cover is mostly based on Griesmeier (2021). Source: Hillshade – Geoland Basemap (http://geoland.at).
5.2 Bucheben
Again, Hottinger (1935) described the Bucheben landslide (Figs. 10f, 11) for the first time. Fellner (1993; unpublished report) made a brief structural analysis of the scarp area with a special focus on present-day block stability. The scarp is developed in Kalkglimmerschiefer and prasinite, which have a dip of 10–15° from west to north (Dieter Fellner, unpublished report). Joints dipping 40–50° towards the east are regarded as the most efficient for the release of the rock mass on the glacially oversteepened slope.
Morphological features indicate a relatively younger rockslide/rock flow (no. 8 in Fig. 11) just below the scarp area which did partly override the Bucheben landslide deposits. The latter can be mapped as two contiguous bodies on both sides of the Hüttwinkl creek. The chapel of Bucheben (1144 m) sits on the eastern landslide mass. The surface is covered by angular boulders made up of predominantly prasinite but also of Kalkglimmerschiefer. Toma hills can be traced 2 km north of Bucheben on top of a valley fill, which was formed before the landslide event as a result of mass movement action before (see Sect. 6).
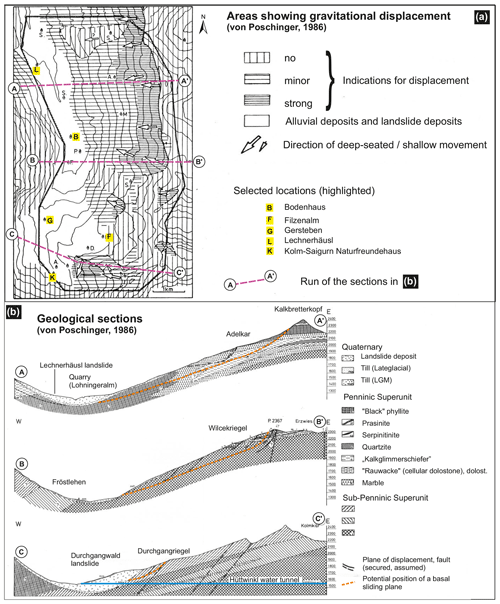
Figure 12(a) Area showing gravitational displacement (after von Poschinger, 1986; terminology translated ). (b) Geological sections after von Poschinger (1986). Location of the sections is indicated in (a). Translation of the legend.
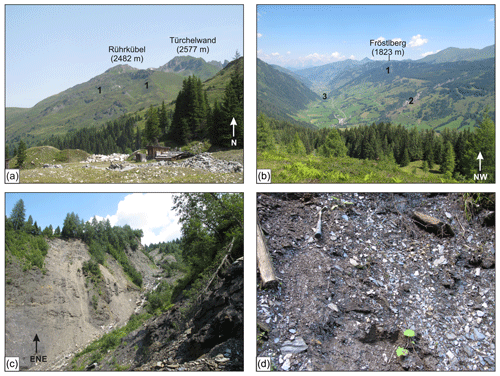
Figure 13Slopes affected by DSGSDs: (a) View from Adelkaralm marble quarry to the western slope of Rührkübel (location in Fig. 2) consisting mostly of Kalkglimmerschiefer and prasinite. Multiple scarps (1) are evident. (b) View from the Felderalm at the scarp of Fröstlberg (1). The flanks of the Teufenbach eroded into disintegrated DSGSDs slopes is evident (2 – site of (c)). (c) Flanks along the Teufenbach in ca. 1200 m a.s.l. (site 2 in (b)) eroded in DSGSDs. Disintegrated and tilted dark phyllite (“Schwarzphyllit”). (d) Further disintegration of dark phyllite due to DSGSD. The schistosity is still recognizable in the lower part (dip 260/20) but the material is extremely weak and shows a transformation to loose debris (hammer for scale).
Like in the case of Lechnerhäusl, an initial rockslide transformed into a rock avalanche whose distal parts disintegrated and moved as isolated toma (no. 5 in Fig. 11). Abele (1974) reconstructed a landslide area of > 1 km2, a travel length of 2.2 km and a Fahrböschung of 25°. According to our reconstructions, we get a contiguous landslide area of 1.5 km2, a travel length of 3.4–3.6 km and a Fahrböschung of 16–17°.
The Bucheben landslide is relatively older than the Lechnerhäusl landslide. A 13.5 m deep drill core at site B (Figs. 3, 11), 500 m upstream of the southernmost landslide deposits and ca. 200 m upstream of the quite small rockfall deposits of Frohnwirt (made up of Kalkglimmerschiefer boulder), helps to constrain the age. 14C dating of wood samples from the lower sandy layer (13.5–8.5 m below the surface), partly rich in organic material, provided ages of ca. 8.6–8.4 ka cal BP for 11.8 m below the surface and ca. 8.2–8.0 ka cal BP for 8.7 m below the surface. This fine-grained layer is topped by coarse gravel followed by a fining-upward sequence with sand and finally silt (4.4–2 m). The 14C dating of wood gave an age of ca. 2.3–2.0 ka cal BP. In the absence of further age constraints, we regard the lower fine-grained deposits to be a result of the damming by the Bucheben landslide which happened before 8.6–8.4 ka at the end of the Early Holocene. According to this hypothesis, the Frohnwirt rockfall caused the formation of the upper backwater deposits and thus occurred in the Late Holocene just before 2.3–2.2 ka.
During the field trip we will stop at Bucheben (1144 m) and study the scarp and deposits of the Bucheben landslide as well as the surrounding landscape (backwater areas, DSGSDs, etc.).
Exner (1957) emphasized mass movements in his geological map of Gastein. There he differentiated between “im Schichtverband abgerutschte Gesteinsmassen” (in the sense of displaced rock masses with a still recognizable set of discontinuities) and “abgerutschte Gesteinsmassen und Bergsturz-Blockwerk” (displaced rock masses and landslide boulders). The first term is covered by what we call “area affected by DSGSD” (see Steinbichler et al., 2019). Exner's (1957) second term has the disadvantage of not discriminating between deposits of catastrophic landslide (e.g., Bucheben) and totally disintegrated rock masses at, e.g., the toe of DSGSDs (see also the geological map in Fig. 1 which follows the same classification). Exner (1957) realized that displaced rock masses with a moderate disintegration exist below an extensive cover of subglacial till some meters thick on the lower parts of the eastern slopes. Thus, he regarded those slope deformations as having formed during an interglacial (in the sense of pre-LGM).
Von Poschinger (1986) made kinematic analyses of the slopes of the eastern flank between Kolm-Saigurn and the Lechnerhäusl landslide deposits based on the set of discontinuities (joints, foliation, schistosity, faults). He showed that only a combination of the westward-dipping main planar fabric and suitable joints enabled the creeping (meaning slow displacement) slope deformation along distinct sliding planes (Fig. 12). On the other hand, he as well realized that the lower parts of the slopes show only slight to moderate indications of slope deformation compared to the upper parts (Fig. 12a). The primary movements, which affected an area of 16 km2 and an estimated total volume of 0.8 to 2.4 km3, probably do not take place along a single defined slide plane but rather along many sliding horizons that cannot be precisely defined. During this process, the rock is broken up into sometimes huge rock slabs with a size of up to some square kilometers, The movements of the valley flank, which can also be classified as a “Talzuschub” (“valley closure”; a bulging toe of a slope due to slope deformation; see Steinbichler et al., 2019), probably began before the LGM and are still continuing today, at least in part. According to von Poschinger (1986), there is currently no risk of the right flank of the upper Hüttwinkl transitioning from (slow) creeping to (accelerated) sliding processes.
Fellner (1993; unpublished report), who investigated the eastern flank north of von Poschinger's study area, emphasized the influence of the abundantly occurring, mechanically weak dark phyllite (Fig. 13c, d) as a condition for the DSGSDs beside the importance of the general dip of the main planar fabric. He classified the DSGSDs as “gleitender Talzuschub” (“valley closure” due to sliding). Mountain rupture phenomena like scarps and tension gaps as well as vertical movements of large rock slabs with the size of a square kilometer with vertical offsets of more than 140 m characterize the uppermost slope areas of the eastern flank. Due to the morphological clarity and freshness of the observed mass movement phenomena and the fact that the numerous tension gaps hardly show any backfilling, Fellner (1993) concludes that there are ongoing movements. Based on the case of the Teufenbach torrent (northeast of Bucheben in Fig. 11; Fig. 13b, c), Baur et al. (1992) emphasize that disintegrated rock masses at the toe of a Talzuschub (DSGSD) are a major source for catastrophic debris flows.
The formation of the backwater deposits south of Einödkapelle (1012 m), which show an onlap on the toe of the Fröstlberg slope (Figs. 11, 13), is of importance for understanding the development of the lower Hüttwinkl Valley (Figs. 2, 3). The Hüttwinkl creek has already incised into this deposit (Figs. 3, 11) and is now already incising into bedrock, but the slightly raised level is still evident upstream. Moreover the backwater level existed already when the Bucheben landslide (Sect. 5.2) occurred, enabling the emplacement of the toma at this valley floor level. Currently there are some options which might have caused this situation. (1) The Fröstlberg ridge is a prominent scarp (Fig. 13a) where the outlines of the displaced mass (in the sense of a DSGSD) below are easily detectable. Like in the cases of DSGSD discussed above, the lower part is covered by till and missing any strong indication of mass movement activity. However, a till cover can be preserved if sliding is the main process. (2) There are clast-supported boulders of up to more than 100 m3 in size (4B in Fig. 11), mainly Kalkglimmerschiefer but also occasionally prasinite. Their source area must be on the western side but not from immediately above the deposit. This sediment could potentially document the most distal part of the Bucheben rock avalanche and/or the product of a larger rockfall from the western side with a so far not identified scarp. (3) There might have been a “valley closure” due to a DSGSD from Fröstlberg and/or a rockfall deposit, maybe also with the contribution of a debris-flow-fed fan from the east, up to the Early Holocene. Such a situation resulted in a slight shift in the river, leading to the formation of an epigenetic valley formed in bedrock which preserved the toe of the Fröstlberg DSGSD from further erosion and hence permanent reactivation.
Additional data are available from the authors upon reasonable request.
JMR and MS conceptualized the field trip and the article together. JMR wrote the major part and made most figures. Geological framework and Fig. 1 are a contribution of MS.
The contact author has declared that neither of the authors has any competing interests.
Publisher’s note: Copernicus Publications remains neutral with regard to jurisdictional claims made in the text, published maps, institutional affiliations, or any other geographical representation in this paper. While Copernicus Publications makes every effort to include appropriate place names, the final responsibility lies with the authors.
The authors thank the following persons for their helpful contributions: Benjamin Huet (on bedrock geology), Michael Lotter (on mass movements), Alfred Gruber (on design of figures) and Marc Ostermann (on the debris flow event of August 2023). Thomas Zwack and Wolfgang Jaritz provided data of the drill core at Frohnwirt (site B). The authors highly appreciate the commitment of the editors of this DEUQUA Special Publications field guide, Bernhard Salcher and Henrik Rother.
We dedicate this work to the memory of two great explorers of the Hohe Tauern mountain range, who inspired us in our research and passed away far too soon: Reinhard Böhm (climate researcher; 1948–2012) and Gerhard Pestal (geologist; 1958–2014).
Abele, G.: Bergstürze in den Alpen, ihre Verbreitung, Morphologie und Folgeerscheinungen, Wissenschaftliche Alpenvereinshefte, 25, 230, https://bibliothek.alpenverein.de/webOPAC/01_Alpenvereins-Publikationen/06_wiss._Alpenvereinshefte/AV-HeftNr.025.pdf (last access: 10 June 2024), 1974.
Agliardi, F., Crosta, G., and Zanchi, A. : Structural constraints on deep-seated slope deformation kinematics, Eng. Geol., 59, 83–102, https://doi.org/10.1016/S0013-7952(00)00066-1, 2001.
Auer, I., Prettenthaler, F., Böhm, R., and Proske, H. (Eds.): Zwei Alpentäler im Klimawandel, in: Alpine Space — Man & Environment, Vol. 11, Innsbruck University Press, Innsbruck, Austria, 199 pp., ISBN 978-3-902719-44-72010, https://www.uibk.ac.at/iup/buch_pdfs/alpine_space_vol.11.pdf (last access: 10 June 2024), 2010.
Baur, M., Edmaier, B., and Spaun, G.: Talzuschübe als Geschiebeherde für Murgangereignisse in Saalbach und Rauris (Land Salzburg), in: Internat. Symp. INTERPRAEVENT 1992, 29 June–3 July 1992, Bern, Switzerland, Verlag Forschungsgesellschaft für vorbeugende Hochwasserbekämpfung, Klagenfurt, 165–180, ISBN 3-901164-03-0, 1992.
Bichler, M. G., Reindl, M., Reitner, J. M., Drescher-Schneider, R., Wirsig, C., Christl, M., Hajdas, I., and Ivy-Ochs, S.: Landslide deposits as stratigraphical markers for a sequence-based glacial stratigraphy: a case study of a Younger Dryas system in the Eastern Alps, Boreas, 45, 537–551, https://doi.org/10.1111/bor.12173, 2016.
Böhm, R., Schöner, W., Auer, I., Hynek, B., Kroisleitner, C., and Weyss, G.: Gletscher im Klimawandel, Vom Eis der Polargebiete zum Goldbergkees, Zentralanstalt für Meteorologie und Geodynamik, Morava, 112 pp., ISBN 978-3-200-01013-0, 2007.
Böhm, R., Auer, I., and Schöner, W.: Labor über den Wolken – die Geschichte des Sonnblick Observatoriums, Böhlau, Wien-Köln, Weimar, 380 pp., ISBN 978-3-205-78723-5, 2011.
Dirnberger, D. and Hilberg, S.: Hydrogeologie im Tauernfenster – Fallbeispiel Rauristal, Salzburg, Grundwasser, 25, 31–41, https://doi.org/10.1007/s00767-019-00442-x, 2020.
Dramis, F. and Sorriso-Valvo, M.: Deep-seated gravitational slope deformations, related landslides and tectonics, Eng. Geol., 38, 231–243, https://doi.org/10.1016/0013-7952(94)90040-X, 1994.
Dufresne, A., Bösmeier, A., and Prager, C.: Sedimentology of rock avalanche deposits – case study and review, Earth-Sci. Rev., 163, 234–259, https://doi.org/10.1016/j.earscirev.2016.10.002, 2016.
Exner, C.: Erläuterungen zur Geologischen Karte der Umgebung von Gastein, Verlag der Geologischen Bundesanstalt, Wien, 168 pp., 1957.
Fellner, D.: Massenbewegungen im Bereich Bucheben im Hüttwinkeltal bei Rauris (Salzburg, Österreich), Jahrbuch der Geologischen Bundesanstalt, 136, 307–313, 1993.
Geologische Bundesanstalt Österreich: Geodaten – Bundesland Salzburg (1 : 200 000), Tethys RDR, Geologische Bundesanstalt (GBA), Wien, https://doi.org/10.24341/tethys.190, 2022.
Griesmeier, G.: Zusammenstellung ausgewählter Archivunterlagen der Geologischen Bundesanstalt 1 : 50 000 – 154 Rauris, Vienna, 2021.
Gruber, F.: Das Raurisertal: Gold – Bergbaugeschichte, Eigenverlag, Marktgemeinde Rauris, 256 pp., 2004.
Hansche, I., Fischer, A., Greilinger, M., Hartl, L., Hartmeyer, I., Helfricht, K., Hynek, B., Jank, N., Kainz, M., Kaufmann, V., Kellerer-Pirklbauer, A., Lieb, G. K., Mayer, C., Neureiter, A, Prinz, R., Reingruber, K., Reisenhofer, S., Riedl, C., Seiser, B., Stocker-Waldhuber, M., Strudl, M., Zagel, B., Zechmeister, T., and Schöner, W.: KryoMon.AT – Kryosphären Monitoring Österreich, 2021/22 Kryosphärenbericht No. 1, Österreich, Bundesministerium Klimaschutz, Umwelt, Energie, Mobilität, Innovation und Technologie, 204 pp., https://doi.org/10.25364/402.2023.1, 2023.
Heim, A.: Bergsturz und Menschenleben, Beiblatt zur Vierteljahrschrift der Naturforschenden Gesellschaft in Zürich, 20, 1–218, 1932.
Hellerschmidt-Alber, J.: Bericht 1997 Über geologische Aufnahmen im Penninikum des Hüttwinkeltales auf Blatt 154 Rauris, Jahrbuch der Geologischen Bundesanstalt, 141, 297–302, 1998.
Hottinger, A.: Geologie der Gebirge zwischen der Sonnblick-Hocharn-Gruppe und dem Salzachtal in den östlichen Hohen Tauern, PhD thesis, Eidgenössische Technische Hochschule Zürich, 123 pp., https://www.research-collection.ethz.ch/bitstream/handle/20.500.11850/134235/eth-20951-02.pdf?sequence=2 (last access: 10 June 2024), 1935.
Ivy-Ochs, S., Monegato, G., and Reitner, J. M.: Chapter 20 – The Alps: glacial landforms during the deglaciation (18.9–14.6 ka), in: European Glacial Landscapes – The Last Deglaciation, edited by: Palacios, D., Hughes, P. D., García-Ruiz, J. M., and Andrés, N., Elsevier, 175–183, https://doi.org/10.1016/B978-0-323-91899-2.00005-X, 2023a.
Ivy-Ochs, S., Monegato, G., and Reitner, J. M.: Chapter 55 – The Alps: glacial landforms from the Younger Dryas Stadial, in: European Glacial Landscapes – The Last Deglaciation, edited by: Palacios, D., Hughes, P. D., García-Ruiz, J. M., and Andrés, N., Elsevier, 525–539, https://doi.org/10.1016/B978-0-323-91899-2.00058-9, 2023b.
Klasen, N., Fiebig, M., Preusser, F., Reitner, J. M., and Radtke, U.: Luminescence dating of proglacial sediments from the Eastern Alps, Quatern. Int., 164, 21–32, https://doi.org/10.1016/j.quaint.2006.12.003, 2007.
Lichtenecker, N.: Die gegenwärtige und die eiszeitliche Schneegrenze in den Ostalpen, in: Verhandlungen der III Internationalen Quartär-Konferenz, INQUA, Vienna, Austria, September 1936, edited by: Götzinger, G., Geologische Landesanstalt, Vienna, Austria, 141–147, 1938.
McSaveney, M. J. and Davies, T. R. H.: Inferences from the morphology and internal structure of rockslides and rock avalanches rapid rock mass flow with dynamic fragmentation, in: Landslides from Massive Rock Slope Failure, edited by: Evans, G. E., Mugnozza, G. S., Storm, A., and Hermanns, R. L., Springer, Dodrecht, 285–304, https://doi.org/10.1007/978-1-4020-4037-5_16, 2006.
Penck, A.: Gletscherstudien im Sonnblickgebiete, Zeitschrift des Deutschen und Österreichischen Alpenvereins, 1897, 52–71, 1897.
Penck, A. and Brückner, E.: Die Alpen im Eiszeitalter. 1. Band: Die Eiszeiten in den nördlichen Ostalpen, Tauchnitz, Leipzig, 393 pp., 1909.
Pestal, G., Hejl, E., Braunstingl, R., Egger, H., Linner, M., Mandl, G. W., Moser, M., Reitner, J., Rupp, C., Schuster, R., and van Husen, D.: Geologische Karte von Salzburg 1 : 200 000, Geologische Bundesanstalt, Wien, 2005.
Reitner, J. M.: Glacial dynamics at the beginning of Termination I in the Eastern Alps and their stratigraphic implications, Quatern. Int., 164–165, 64–84, https://doi.org/10.1016/j.quaint.2006.12.016, 2007.
Reitner, J. M.: The Imprint of Quaternary Processes on the Austrian Landscape, in: Landscapes and Landforms of Austria, edited by: Embleton-Hamann, C., Springer International Publishing, Cham, 47–72, https://doi.org/10.1007/978-3-030-92815-5_3, 2022.
Reitner, J. M., Ivy-Ochs, S., Drescher-Schneider, R., Hajdas, I., and Linner, M.: Reconsidering the current stratigraphy of the Alpine Lateglacial: Implications of the sedimentary and morphological record of the Lienz area (Tyrol/Austria), E&G Quaternary Sci. J., 65, 113–144, https://doi.org/10.3285/eg.65.2.02, 2016.
Schmid, S. M., Fügenschuh, B., Kissling, E., and Schuster, R.: Tectonic map and overall architecture of the Alpine orogen, Eclogae Geol. Helv., 97, 93–117, https://doi.org/10.1007/s00015-004-1113-x, 2004.
Schuster, R., Daurer, A., Krenmayr, H. G., Linner, M., Mandl, G. W., Pestal, G., and Reitner, J. M.: Rocky Austria. Geology of Austria – brief and colourful, Geological Survey of Austria, Vienna, 80 pp., ISBN 978-3-85316-078-7, 2014.
Steinbichler, M., Reitner, J., Lotter, M., and Steinbichler, A.: Begriffskataloge der Geologischen Landesaufnahme für Quartär und Massenbewegungen in Österreich, Jahrbuch der Geologischen Bundesanstalt, 159, 5–49, 2019.
van Husen, D.: Die Ostalpen in den Eiszeiten, Veröffentlichungen der Geologischen Bundesanstalt, Vienna, 2, 1–24, ISBN 978-3-900312-58-9, 1987.
van Husen, D. and Reitner, J. M.: Quaternary System, in: The lithostratigraphic units of Austria: Cenozoic Era(them), edited by: Piller, W. E., Abhandlungen der Geologische Bundesanstalt, Vienna, 76, 240–267, ISBN 978-3-903252-14-1, 2022.
von Poschinger, A.: Instabile Talflanken in Kristallingesteinen und ihre geologischen Ursachen, dargestellt am Beispiel des oberen Hüttwinkeltales (Land Salzburg, Österreich), PhD thesis, Technische Universität München, 170 pp., 1986.
Walker, M., Head, M. J., Berkelhammer, M., Björck, S., Cheng, H., Cwynar, L., Fisher, D., Gkinis V., Long, A., Lowe, J., Newnham, R., Rasmussen, S. O., and Weiss, H.: Formal ratification of the subdivision of the Holocene Series/Epoch (Quaternary System/Period): two new Global Boundary Stratotype Sections and Points (GSSPs) and three new stages/subseries, Episodes 2018, 41, 213–223, https://doi.org/10.18814/epiiugs/2018/018016, 2018.
Wirsig, C., Zasadni, J., Christl, M., Akçar, N., and Ivy-Ochs, S.: Dating the onset of LGM ice surface lowering in the High Alps, Quaternary Sci. Rev., 143, 37–50, https://doi.org/10.1016/j.quascirev.2016.05.001, 2016.
- How to cite
- Abstract
- Introduction
- General overview and geological setting
- Landscape evolution of the Hüttwinkl Valley since the Last Glacial Maximum (LGM) and before
- Kolm-Saigurn
- Landslides north of Bodenhaus
- Deep-seated gravitational slope deformation (DSGSD) and related deposits
- Data availability
- Author contributions
- Competing interests
- Disclaimer
- Acknowledgements
- References
- How to cite
- Abstract
- Introduction
- General overview and geological setting
- Landscape evolution of the Hüttwinkl Valley since the Last Glacial Maximum (LGM) and before
- Kolm-Saigurn
- Landslides north of Bodenhaus
- Deep-seated gravitational slope deformation (DSGSD) and related deposits
- Data availability
- Author contributions
- Competing interests
- Disclaimer
- Acknowledgements
- References