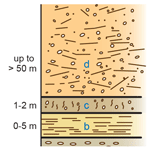
Problems of terrace formation
Paul Woldstedt
- Article
(1808 KB) - Full-text XML
- BibTeX
- EndNote
Woldstedt, P.: Problems of terrace formation, DEUQUA Spec. Pub., 3, 35–40, https://doi.org/10.5194/deuquasp-3-35-2021, 2021.
Special issue statement. This article is part of a special issue published on the occasion of the 70th anniversary of E&G Quaternary Science Journal (EGQSJ). The special issue celebrates the journal's notable contribution to Quaternary research by revisiting selected milestone articles published in the long history of EGQSJ. The German Quaternary Association (DEUQUA) presents translations of the originals and critical appraisals of their impact in tandem anniversary issues of DEUQUASP and EGQSJ, respectively.
Original article: https://doi.org/10.3285/eg.02.1.04
Tribute: https://doi.org/10.5194/egqsj-70-217-2021
Translators: Clare Bamford, Markus Fuchs, Jim Rose and Henrik Rother
Most larger rivers in Central Europe are accompanied by a whole system of higher terraces. Are these terraces, unless considered to be formed by tectonic processes, of glacial or interglacial age? This is the old controversy that has been dealt with in many writings and by numerous authors. Terraces formed by gravel and sand occurring in direct connection to terminal moraines are excluded from consideration here, since it is not disputed by anyone anymore that they are of glacial origin. But what about those terraces that do not derive from tectonic or glaciofluvial processes?
In Germany, mainly under the influence of W. Soergel (cf. especially Soergel, 1921, 1925, 1939), it has generally been accepted that all terraces formed in a glacial climate. Furthermore, the concept implies that they originated during the advance phase of a glaciation. This is supported by a variety of observations: the occurrence of a distinct cold fauna (with Elephas primigenius, Rhinoceros tichorhinus, Ovibos moschatus, etc.) in the upper parts of the terraces, a connection to glaciofluvial deposits, and finally the burial of these terraces by glacial deposits and laminated clays. Since the classical investigations of Siegert and Weissermel (1911) in the Saale area, this model has been applied to numerous other areas, such as Upper Saxony by Grahmann (1925).
However, for a long time terraces have also been known, in which only a warm fauna was found. Siegert and Weissermel (1911) found a terrace in the Saale area which appears to be younger than the Elster glaciation but older than the so-called Main Terrace of that region. This terrace was called the “Higher First Interglacial Terrace” and contains Elephas antiquus, without any cold-loving fauna. Thus, Siegert and Weissermel (1911) saw this terrace as a true interglacial deposit, into which the river later incised and then deposited the Main Terrace in the second half of the interglacial period (formation of the Main Terrace). However, W. Soergel notes that the older terrace with thermophilic Elephas antiquus fauna, also occurring in the Ilm, Gera and Unstrut areas, is an equivalent of a less pronounced glacial period (Pre-Riss) whose impact did not go so far as to drive the warm-adapted animals away completely.
This idea, however, leads to difficulties. It is not possible that glacial-climatic gravel formations accumulated in the low mountain ranges, while only a few hundred metres lower, a proper interglacial fauna was still present. But other observations are also relevant. For example, the Main Terrace, whose higher parts undoubtedly accumulated under glacial conditions, are not uniform in this respect. They often show a lower part containing a warm fauna. Siegert and Weissermel (1911) mention Elephas antiquus and Rhinoceros mercki1 from the Main Saale Terrace of Uichteritz. Barner (1941) also found the latter mammals in the corresponding Leine Terrace near Gronau, where they were recovered together with a hand-axe of the Middle Acheul. Now these bone remains could of course have been redeposited – the animals lived in the interglacial period itself, while their bones were later washed into the lower part of the terrace during the glacial period deposition. This is undoubtedly possible.
This idea becomes difficult to maintain, however, when Corbicula fluminalis appears in large numbers at locations which surely preserve in situ site conditions. This is indicated by the Unstrut Main Terrace near Körbisdorf (Siegert and Weissermel, 1911, p. 152) and was also reported by Mertin (1940) from the lower section of the Salzke Main Terrace near Köchstedt. Together with Corbicula and Emys orbicularis, the European pond turtle, is also an undisputedly warmth requiring species. At another location on the same Main Terrace, even slightly higher in the profile, Belgrandia marginata was found in a marl deposit (“Schneckenmergel”). Without doubt, we can still expect fully interglacial conditions here as well. However, this cannot be reconciled with the idea that all depositional terraces represent glacial-climatic deposits, as argued by Soergel who considers cold-climatic conditions to be the exclusive driver for the formation of our depositional terraces. Thus, we must accept that there exists a Main Terrace, the lower part of which contains a fully interglacial fauna, whereas the upper part was deposited under glacial conditions.
Whereas a warm-adapted fauna can be found in the basal portion and a cold-adapted in the upper parts of our terraces, the opposite was the case when individual terraces of the Thames were investigated. According to Sandford (1924; cf. also Arkell, 1947), the so-called “Summertown-Radley-Terrace” of the Thames, which lies about 7–8 m above the river near Oxford, contains a cold fauna with Elephas primigenius and Megaceros in the base layers, but a warm fauna (Hippopotamus, Cervus elaphus, Corbicula fluminalis, etc.) in its upper main part. From the corresponding terrace of the lower Thames area near London, the “Upper Flood-plain Terrace”, King and Oakley (1936) indicate peat with Betula nana and Salix lapponum from the base, whilst Zeuner (1945) reports Elephas antiquus and Hippopotamus appear in the upper part.
So here the building up of gravel began under cold conditions and ends under warm ones – just the opposite of the Main Terrace of the Saale area. The solution of this mystery of the formation of the terraces of the Lower Thames was, however, that they are related to eustatic sea level fluctuations. With the rise of the sea level after the glaciation, the river began to accumulate, first in its lower reaches, but later also in its middle reaches, as the Summertown-Radley Terrace near Oxford shows.
Other terraces along the Thames seem to only feature a “warm” part. Especially the Boyn-Hill-Terrace in the London area, which was placed in the Mindel-Riss interglacial period, and the Hanborough-Terrace near Oxford, which is probably of the same age.
Mainly warm faunas, but often with a basal cold zone, are also found in the terraces along the Somme, which have already been described several times. In these terraces, the actual gravel body can be further distinguished from the surface layers that were partly formed under periglacial conditions (cf. see the summary by Zeuner, 1945, 1950).
We are also familiar with these interglacial gravel deposits from our rivers, e.g., the Elbe. According to Grahmann (1931), the last glacial Lower Terrace of the Elbe was increasingly eroded towards the lower reaches during the late glacial period, with erosive amounts in the south of 10–15 m but at least 20 m in the estuary region. Then a new accumulation phase began, which deposited gravel and sands in the southern section (middle reaches) while further downvalley sand deposition dominated. These often contain oak trunks, indicating that the age of this deposit is essentially Atlantic.
According to Horn (1912), terraces in the Hamburg area preserve an approximately 1 m thick layer of gravel within this younger accumulation positioned at about 12 m (a.s.l.), which contain Hirschhorn artefacts of the Kjökkenmöddinger period. The age of this layer can be determined to be about 4000 BC. Further down the valley there are marine formations with Scrobicularia piperata, Cardium edule and Tellina baltica. Above this follow marine muds which were evidently deposited in a brackish environment.
From what has been said, it is clear that along most of the Elbe River, we have a young Holocene, i.e. warm period deposits, with an average thickness of 10 m in the middle reaches which increases in thickness towards the lower reaches. The further north-west, the difference in altitude between the Lower Terrace and the Holocene deposit becomes smaller. In the Elbe estuary, the Lower Terrace is partly at the level of the Holocene fill and partly dipping below it.
The Holocene accumulation of the Elbe is apparently related to the post-glacial rise in sea-level in the North Sea. This effect is not entirely restricted to the lower reaches of the Elbe, but appears to be felt quite far upstream along the river. This is therefore an example of a true warm period, i.e., “interglacial” accumulation of sediment at least up to the middle reaches. The “pumice terrace”, which was evidently built up after the Allerød period, can be regarded as a corresponding formation in the Lower Rhine area. In the Weser and Ems areas, too, we can expect accumulations of the same age, but these have not yet been identified.
Zeuner (1945) describes such accumulations associated with a sea-level rise as “thalassostatic”. Certain terraces in the Thames and Somme also belong to this category. In the case of the Thames, both the last interglacial terrace (Summertown-Radley-T.) and the Mindel-Riss interglacial terrace (Hanborough-Terrace) extend at least to the middle reaches.
The height of these deposits in the estuary and the extent of accumulation, was probably mainly due to the length of the interglacial period concerned and the specific amount of the sea level rise. The above-mentioned “Higher First Interglacial Terrace” of the Saale River of Siegert and Weissermel (1911) may be regarded as such a gravel deposit formed in the long Mindel-Riss interglacial period, which moved up valley from the estuary. It would then be of about the same age as the Hanborough (or Boyn Hill) terrace of the Thames area.
The question now arises as to how a rise in sea level can cause a river to aggrade. Because the physical forces within the river regime, which are initially solely responsible for accumulation or incision, are, as it seems at first glance, not changed by this.
But this should be the case if the sea level rises relatively quickly. The situation is different if the sea-level rise is very slow or has come to a standstill. A river with a balanced gradient will push a delta into the sea if the sea level remains constant (cf. Fig. 1 after Quiring, 1926). However, this will gradually reduce the gradient in the estuary and the backfill area will gradually move up-valley inland from the estuary. The physical forces within the river regime change to the extent that the transport force decreases as a result of the lower gradient, in a wave-like manner that gradually moves upstream from the estuary.
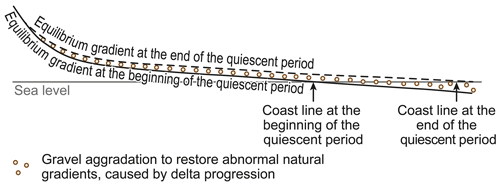
Figure 1Schematic drawing of a fluvial aggradation wedge propagating upstream from the river mouth at constant sea level (after Quiring, 1926).
The same will be the case, albeit to a lesser extent, if the sea level rises only slowly and the accumulation in the estuary (delta formation etc.) is stronger. In this case, although to a lesser extent than in the case of the sea-level rise considered above, a (weaker) wave of sediment deposition will continue upstream from the estuary.
As mentioned above, the main accumulation along the Holocene Elbe River occurred during the Atlantic period and later. By this time, however, the main post glacial rise in sea level had long been completed. Of the 90–100 m by which the ocean level was lower during the maximum of the last glaciation, the level had risen by at least 70–80 m at the beginning of the Atlantic period. The rise of the sea level was very rapid at first, it then slowed down considerably, and the accumulation of sediment apparently began in connection with this.
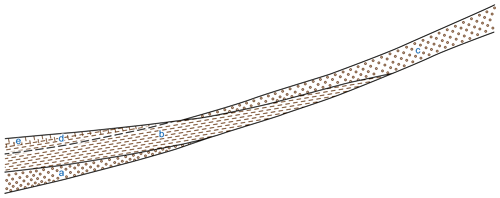
Figure 2Schematic drawing of aggradation processes over the course of a glacial – interglacial cycle. a = “Cold” eustatic gravel aggradation within the lower valley reaches at low sea level. b = `Warm” eustatic gravel aggradation at rising and/or constant sea level. c = Glacial aggradation at the onset of the next glaciation. d = Erosion within the lower valley reaches with renewed fall in sea level. e = Periglacial modification of interglacially aggraded gravels.
The views developed in the previous section are presented schematically in the longitudinal river profile shown in Fig. 2. In the lower reaches, sedimentation began during the still relatively cold phase associated with the downwasting of the ice masses. Sedimentation intensifies during the warm period, with interbedded marine layers intervening more frequently in the estuary. At the end of the warm period, a new erosional impulse will take hold here when the ocean level drops again. The surviving remnants of the warm period sedimentation usually also show signs of a switch to a periglacial climate at their surface.
Further up in the longitudinal river profile, the main amount of accumulation derived from sedimentation during warm periods. Occasionally the stratigraphically deepest parts may still be from cold-periods, as the example of the Summertown-Radley Terrace of the Thames shows. The portion of accumulation associated with warm-phase sedimentation decreases in the upstream direction and will increasingly be overlain by cold-climate gravels, which accumulated during the following glacial stage. Eventually this material forms the entire gravel body.
Thus, profiles from different parts of the river course have a very different appearance depending on where they are located. Also, the sequence of events need not be as simple as has been assumed here. In the Saale area, as already mentioned, a double gravel formation occurred during the Mindel-Riss interglacial period. The first cycle only went as far as the formation of a “warm” accumulation. Then – for reasons not yet understood – a period of incision occurred again, whereupon a new accumulation phase started, first under warm, then under cold climate conditions.
The longitudinal river profile shown in Fig. 2, which attempts to summarise the eustatic and glacial-climatic terracing concept, is, it must be emphasised again, a purely schematic diagram. It does not consider the deeper incision of rivers related to the continuous lowering of interglacial sea levels during the Quaternary period. Also, the schematic diagram does not cover all cases occurring in nature. There is no doubt that in reality we also encounter purely glacial accumulations which lack any contribution from warm-period sedimentation. This includes most – but not all – of the so-called Lower Terraces of our rivers. In the Elbe region, for example, the Lower Terrace from the upper reaches to the estuary, where the latter becomes submerged under Holocene deposits, is an ice-age accumulation. In the case of the ice age River Elbe the influx of material derived from areas of periglacial erosion and of glacio-fluvial material from the actual ice margin – the Elbe was a first order glacial valley during last glaciation – was so great that aggradation filled up the whole length of the river. Unfortunately, we are missing the record of the lower reaches of the Elbe at that time, which we have to imagine as extending about 500 km further into the North Sea.
We are indebted to F. Zeuner for a detailed study of the lower Thames area, taking into account the important investigations of Woolridge (1938). He points out a number of Thames terraces (Zeuner, 1945, p. 114ff), which differ from others due to their steeper gradient of both their base and surface. These include, for example, the “Higher Gravel Train”, which possibly belongs to the Glacial Period, and the terrace known as the “Kingston-Leaf”, which perhaps belongs to the Mindel glacial period. It is apparent that both are related to a low sea level and that these terraces formed during glacial times.
A glacial-stage deposit of the age of the “Lower Terrace” of most Central European rivers, i.e., a Würm glacial age, is apparently the “Lower Floodplain Terrace” of the lower Thames area (Northmoor Terrace of the Oxford area). F. Zeuner places it into the Würm I/Würm II interstadial accompanied by a relatively high ocean level. However, nothing is known of such a high ocean stand within the Würm Glaciation. Thus, this “Lower Terrace” most likely derives from a periglacial fill formed during a glacial period similar to those occurring in the Elbe region.
The question is whether there is not also a corresponding deposit of the Riss Glacial in the Thames area. This could be the Taplow Terrace of the lower Thames area, which corresponds to the Wolvercote Terrace of the Oxford area. Both deposits lack a distinctive warm fauna. The Wolvercote Terrace of the Oxford area has indeed been considered glacial by several researchers.
If the concept of (at least) four glacial depositional sequences is correct, the following schematic is derived for the Thames area:
Accordingly, we observe a regular alternation of cold- and warm-period deposits, whereby the glacial deposits were laid down at low sea level, and the interglacial deposits at a high sea level. In interglacial times, a depositional wedge formed downstream and migrated upstream. Glacial deposits, on the other hand, start accumulating in the upstream reaches and migrate downwards.
In the literature, one often finds the idea that a stronger gravel deposition occurred in the rivers of the low mountain ranges “under the damming influence of the advancing ice”. Grupe (1912, 1926) was the main proponent of this view, pointing to the Weser terraces and assuming considerable aggradational thicknesses.
However, as already Soergel (1925) pointed out, in no way could a “damming-up” by the advancing ice have longer distance effects regarding the regular pattern of river aggradation. The influence of the ice only becomes noticeable when valleys are closed off completely and pro-glacial lakes form in front of the ice margin into which the rivers now flow. This, however, cannot no longer be considered as a normal pattern of fluvial gravel deposition as the river now begins to build a delta into the reservoir, while laminated clays are deposited into the deeper parts of the basin.
Delta formations differ fundamentally from regular terrace fill sequences due to their distinctive bedding (foreset and topset beds). It is curious that so far only very few examples of genuine delta formations have been reported from the valleys of our low mountain ranges as we should expect them on a much wider scale.
The Weser valley between Minden and Hameln can be used here as an example of such a lake which formed as the valley was dammed up by the advancing ice. In this area we find everywhere a typical sequence of strata shown in Fig. 3 (cf. Explanatory Notes to the Geological Sheets 1 : 25 000). This begins at the base with gravels of the Weser Middle Terrace, which features an average thickness of 12–15 m. Above it follow horizontally bedded laminated clays with a thickness of up to 5 m, whereby the thickness generally decreases in the upstream direction. The laminated clay is overlain by 1–2 m thick ground moraine of the Saale glaciation. Above this, there are (in places more than 50 m) thick fills of Nordic-sourced material, which was deposited in gaps between the ice by meltwater (kame formations).
The depositional history of this section can be derived from the sediments. After deposition of the Middle Terrace gravel deposits, an ice-dammed lake formed in the area between Porta Westfalica and Hameln. This can only have occurred because a glacier tongue of the Saalian ice sheet advancing southward through the gap at Porta Westfalica, damming the valleys east of it at least as far as Hameln. The Weser flowed into the southeast of this reservoir. Its delta formations developed sequentially and probably progressively southwards. Laminated clays were deposited in the reservoir itself. If water from the reservoir, i.e., ultimately Weser water, overflowed to the west, it can no longer be identified as Weser water, as it no longer transported Weser gravels, but only rocks eroded from its channel after its overflow.
The ice, which had initially only penetrated through the Porta Westfalica Gap, eventually also crossed the Weser uplands in a broad front and covered the deposits of the Rinteln pro-glacial lake with its ground moraine. After the Saale ice had reached its probable relatively short-loved maximum, which was located at the southern edge of the Münster lowlands and in the Lower Rhine area, it disintegrated into extensive masses of dead ice. Such masses also filled the Weser valley between Bad Oeynhausen and Hameln. Sands, gravels and boulders were washed into these gaps by meltwater from the active ice which by then was located directly north of the Weser-Wiehengebirge.
In most cases, we can imagine the effects of the advancing ice to be similar. Only relatively rarely did the rivers simply shift their course, nor did the normal river aggradation increase under the damming influence of the ice. Instead, in most cases, reservoirs formed with deltaic bodies building into them and the deposition of laminated clays. Yet, often it is not possible to trace a low mountain range river with its characteristic gravel signature across these phases of ice fluctuation because it cannot be determined where the water overflowing and leaving the reservoir originally came from.
Gravel aggradation and erosion, i.e., terrace formation, can, as we have seen, be triggered by changes in climate as well as eustatic factors. But there is a third possibility: tectonics. There can be no doubt that tectonic uplift and subsidence influences the river regime and thus can lead to changes in deposition or erosion. Numerous authors have assumed a tectonic origin for the Rhine terraces. Quiring (1926) in particular has advocated this point of view. According to him, the West German block (“Großscholle”) is undergoing a tilting movement interrupted by quiescent periods, which raises the south-eastern part and lowers the north-western part, i.e., the Netherlands. According to Quiring, in this scenario the terraces formed during the quiescent periods when the tilting was interrupted or even somewhat regressive. During these periods the Rhine pushed its delta seaward in the form shown in Fig. 1, and the reduction of the gradient in the estuary area led to an aggradational wedge migrating upstream. According to Quiring, the phases of tectonic pause and movement, and thus the formation of terraces, have nothing to do with the ice ages. In his view, the older quiescent and terrace formation periods coincide with interglacial periods. However, according to the same author the formation of the “Lower Terrace”, which corresponds to a period of reduced tectonic activity, falls into an ice age – his Solutré Ice Age – while the formation of the pumice-bearing youngest terrace of the Rhine River coincides with a tectonically quite and climatically warmer period. What has not been considered by Quiring, however, are the eustatic fluctuations in sea level and the glacial-climatic gravel sequences. Both undoubtedly play an important role in the Rhine area, thus there is an interplay of all three factors: the glacial-climatic, the eustatic and the tectonic.
The Central European river terraces have so far been considered too one-sided and only from the point of view of glacial-climatic gravel formation. In this regard, revisions will be necessary and in the future we must decide in each case of an individual fill: is it glacial-climatic, is it eustatic or is it of tectonic origin? The explanations given above offer more problems than solutions. They are only intended to prompt the further pursuit of the matter.
This paper was edited by Markus Fuchs.
Arkell, W. J.: The Geology of Oxford, 1st edn., Oxford Clarendon Press, Oxford, UK, 267 pp., 1947.
Barner, W.: Altpaläolithische Funde aus dem südlichen Hannover, Quartär, 3, 44–55, 1941.
Grahmann, R.: Diluvium und Pliozän in Nordwest-Sachsen, Abhandlungen der Mathematisch-Physischen Klasse der Sächsischen Akademie der Wissenschaften, 39, 4, Leipzig, Germany, 1925.
Grahmann, R.: Das Stromgebiet der Elbe, in: Handbuch der vergleichenden Stratigraphie Deutschlands, Alluvium, Berlin, Germany, 206–214, 1931.
Grupe, O.: Die Flußterrassen des Wesergebietes und ihre Altersbeziehungen zu den Eiszeiten, Zeitschrift der Deutschen Geologischen Gesellschaft, 64, 265–298, 1912.
Grupe, O.: Tal- und Terrassenbildung im Gebiet der Werra-Fulda-Weser und Soergel's “Gliederung und absolute Zeitrechnung des Eiszeitalters”, Geol. Rundsch., 17, 161–196, 1926.
Horn, E.: Die geologischen Verhältnisse des Elbtunnels nebst einem Beitrage zur Geschichte des unteren Elbtales, Jahrbuch der Hamburgischen Wissenschaftlichen Anstalten, 29, 35–58, 1912.
King, R. and Oakley, K. P.: The Pleistocene Succession in the lower parts of the Thames Valley, P. Prehist. Soc., 2, 52–76, https://doi.org/10.1017/S0079497X00021666, 1936.
Mertin, H.: Das erstinterglaziale Vorkommen von Corbicula fluminalis bei Köchstedt westlich Halle a. d. Saale, Zeitschrift für Geschiebeforschung und Flachlandsgeologie, 16, 53–81, 1940.
Quiring, H.: Die Schrägstellung der westdeutschen Großscholle im Känozoikum in ihren tektonischen und vulkanischen Auswirkungen, Jahrbuch der Preußischen Geologischen Landesanstalt zu Berlin, 47, 486–558, 1926.
Sandford, K. S.: The river-gravels of the Oxford District, Quarterly Journal of the Geological Society, 80, 113–170, https://doi.org/10.1144/GSL.JGS.1924.080.01-04.09, 1924.
Siegert, L. and Weissermel, W.: Das Diluvium zwischen Halle a. S. und Weißenfels, Abhandlungen der Königlich preussischen geologischen Landesanstalt Neue Folge, 60, Berlin, Germany, 1911.
Soergel, W.: Die Ursachen der diluvialen Aufschotterung und Erosion, Fortschritte der Geologie und der Paläontologie, 5, Berlin, Germany, 1921.
Soergel, W.: Die Gliederung und absolute Zeitrechnung des Eiszeitalters, Fortschritte der Geologie und der Paläontologie, 13, 125–251, 1925.
Soergel, W.: Das diluviale System, Die geologischen Grundlagen der Vollgliederung des Eiszeitalters I, Fortschritte der Geologie und Paläontologie, 39, 155–292, 1939.
Toepfer, V.: Die glazialen und präglazialen Schotterterrassen im mittleren Saaletal, Ber. nat. forsch. Ges. Freiburg i. Br., 32, 1–106, 1933.
Wooldridge, S. W.: The glaciation of the London Basin and the evolution of the lower Thames drainage system, Quarterly Journal of the Geological Society, 94, 627–668, https://doi.org/10.1144/GSL.JGS.1938.094.01-04.21, 1938.
Zeuner, F. E.: The Pleistocene Period: Its Climate, Chronology and Faunal Succession, Antiquity, 20, 52–53, https://doi.org/10.1017/S0003598X00019323, 1945.
Zeuner, F. E.: Dating the Past. An Introduction to Geochronology, 2nd edn., Methuen & Co. Ltd., London, UK, 474 pp., 1950.
Toepfer (1933) places this terrace remnant of Uichteritz, however, not to the main terrace, but to its 2nd glacial terrace, which is supposed to correspond to the first Saale advance (Riß I). In principle, this does not change the opinion expressed here, since in both cases it is a matter of gravel aggradation, which is supposed to be formed glacial-climatically, i.e. during the advance of a glaciation.