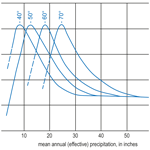
Morphodynamic activity and stability phases instead of pluvial and interpluvial times
Heinrich Rohdenburg
- Article
(2551 KB) - Full-text XML
- BibTeX
- EndNote
Rohdenburg, H.: Morphodynamic activity and stability phases instead of pluvial and interpluvial times, DEUQUA Spec. Pub., 3, 101–110, https://doi.org/10.5194/deuquasp-3-101-2021, 2021.
Special issue statement. This article is part of a special issue published on the occasion of the 70th anniversary of E&G Quaternary Science Journal (EGQSJ). The special issue celebrates the journal's notable contribution to Quaternary research by revisiting selected milestone articles published in the long history of EGQSJ. The German Quaternary Association (DEUQUA) presents translations of the originals and critical appraisals of their impact in tandem anniversary issues of DEUQUASP and EGQSJ, respectively.
Original article: https://doi.org/10.3285/eg.21.1.07
Tribute: https://doi.org/10.5194/egqsj-70-243-2021
Translators: Clare Bamford, Norm Catto, Markus Fuchs and Henrik Rother
Over the course of the Quaternary, all climatic zones around the world were marked by an alternation of extensive erosion (periods of morphodynamic activity) and soil formation (periods of morphodynamic stability). The Holocene represents a period of morphodynamic stability, due to dense vegetation in areas of abundant rainfall, combined with insufficient or evenly distributed rainfall in areas with reduced vegetation. Thus, the ecological conditions characteristic for phases of morphodynamic activitiy cannot be studied or fully understood using an actualistic approach alone. The Pleistocene conditions of morphodynamic activity prevailing in the tropical and subtropical areas must necessarily be due to less evenly distributed rainfall, as only this can explain both less dense vegetation and a substantially increased ratio of surface runoff. However, the question as to whether this also involved a change in the absolute amount of rainfall is of secondary importance: it is likely that rainfall was lower in areas covered with dense vegetation and greater in areas of great aridity than at present. The time-honoured pluvial concept must be abandoned, since in the case of the semiarid and arid areas, it lumps together all of the alleged indications of a former increase (often, however, only of a different type) in the effects of precipitation without ever distinguishing between improved soil water balance on the one hand and an increase in slope erosion produced by a substantially higher ratio of surface runoff on the other. It is these two possibilities, leading to changes in the water balance, that result in the essentially different ecological states of morphodynamic activity and stability, which soil stratigraphy has revealed are never coeval in either the tropical or subtropical areas.
In the early days of geomorphology, it was believed that erosion, albeit not entirely climate-independent, occurred more or less continually across all climatic zones and was primarily controlled by tectonics. Today, the climate dependence of erosion is recognised to a much greater extent with one of the most important results of the climato-genetic geomorphology being the recognition of periods of climate-induced erosional quiescence, even in uplifted areas with a strong relief. The idea of a climate-induced alternation between periods of erosion quiescence and periods of strong erosion is particularly plausible for Central Europe. On the one hand, the natural mixed deciduous forests of the Pleistocene interglacials and the Holocene, – being considered natural only in areas without anthropogenically disturbed vegetation – guarantees good soil protection from erosion. On the other hand, during the Pleistocene glaciations, a different erosion regime prevailed, due to a periglacial climate with strong frost dynamics, the effectiveness of which was manifested by a largely patchy vegetation cover.
The situation is different outside the periglacial areas of the Pleistocene, i.e., in the subtropics and tropics. For a long time, the old idea of continuous formation was maintained for these areas, and is still maintained today in some cases. As a result, relief formation and differentiation in the lowlands of the subtropics and tropics is still commonly related only to the present climatic conditions. According to the proponents of this view, a climato-genetic approach to this question appears unproductive; therefore, the lack of an altitudinal differentiation of relief within these zones, which, at least in part, is even more pronounced than in Central Europe, must be explained tectono-genetically. For the context considered here, it is important to note that, in contrast to Central Europe, many of these zones show no evidence for periods of temporary quiescence of erosion, neither at the present nor in earlier times. Exceptions, however, are the foothills of mountains (and of course the mountains themselves), which in the Pleistocene featured large areas with periglacial environments, and to which a climato-genetic concept has long been applied because of their proximity to the mountain regions. In particular, the formation of pediments in the subtropical dry areas was long seen from this point of view only.
Today, however, it is clear to all geomorphologically oriented soil scientists, as well as to all geomorphologists informed about soil science, that everywhere on Earth, including the wet-dry and humid tropics (e.g., Fölster, 1964, 1969; Rohdenburg, 1969), there have been climate-induced changes in landscape dynamics which were just as pronounced as in Central Europe. Everywhere, periods of strong slope formation alternated with periods of quiescence or limited erosion. In the Mediterranean area, ancient debris-covered slopes have been known for a long time, even at low altitudes (e.g., Mensching, 1955; Wiche, 1961; Butzer, 1964a, b; Rohdenburg and Sabelberg, 1969a, b). In addition, pediplains (“Fußflächen”) or stepped pediplains (“Fußflächentreppen”) also occur at the foot of low mountains, whose formation cannot be attributed to the influence of the neighbouring periglacial altitudinal zonation (Wiche, 1959, 1961; Mensching, 1964a). These debris-covered slopes and lowland pediments are generally explained by assuming a wetter (“pluvial”) climate in which stronger erosion was effective. Evidence of a formerly wetter climate is also seen in past lake highstands, which occurred apparently contemporaneous with major glaciation in the mountains and the formation of slope debris and pediments in the lowlands (e.g., Coque, 1962; Mensching, 1964b).
In the tropics, there are also widespread indications showing that erosion was much greater in the past, these include stepped pediments (“Pedimenttreppe”), debris horizons, stone pavements (“Steinsohlen”), and river beds that were much wider in ancient times than at present (Rohdenburg, 1969, 1970). Both in South America (Bigarella, 1966; Mabesoone, 1966) and in Africa (among others Brückner, 1955; Fölster, 1964, 1969; de Ploey, 1965; Rohdenburg, 1969, 1970; Tricart, 1965; Vincent, 1966; Vogt, 1966), these phases of strong erosion are interpreted as periods of a much drier climate with less dense vegetation than today1, since the present vegetation is not compatible with strongly effective erosion that removes more than just fine material. It must be emphasised that in this respect there are no significant differences between areas with rainforest and those with dry savanna (“Trockenwald”), at least as far as West Africa is concerned.
This overview shows that the independently developed palaeoclimatic conceptions of the subtropics on the one hand and the tropics on the other are contradictory. It is striking that in the works concerning the subtropics, hardly any ideas have been developed about the vegetation cover during times of strong slope erosion. In one of the few statements on this subject, Wiche (1961, p. 148) states that the pluvial forest could not have been denser than that present, but above all he believes that the pluvial forest was not characterized by an extensive ground vegetation cover. Butzer (1961) also does not expect a denser vegetation than today, despite the assumed increase in precipitation, stating that he considers the present vegetation types of the Garrigues and the “light oak woodlands” with “incomplete soil protection” to be the natural vegetation. However, if one considers that these vegetation types have emerged from forests with much better soil protection due to a strong anthropo-zoogenic influence, then Wiche and Butzer should have argued for a thinning for the “pluvial-period” vegetation compared to today's natural vegetation. However, this can no longer be explained by the assumption of a “pluvial-period” increase in precipitation.
Butzer, however, has listed a supposed indication for relatively humid conditions during the deposition of the slope sediments, namely their frequent intercalation with laminated CaCO3 crusts (“Kalkkrustenlamellen”), which he considers to have developed as a sinter-like deposit from a time with increased spring discharge. However, this argument for an increase in precipitation is cannot be valid because the laminated CaCO3 crusts were not formed during sedimentation, but developed subsequently within these sediments as parts of a multilayered Ca-horizon from a higher surface and during a time of intense soil formation (Rohdenburg and Sabelberg, 1969a, b2). Also, the higher lake levels are not clear indicators of higher precipitation, because as Haude (1969) was able to demonstrate using the Dead Sea as an example, the lake level would have to rise considerably in cooler periods even if the precipitation rate remained the same, because evaporation was greatly reduced as a result of the lowering of temperature (cf. Frenzel, 1967). However, if one assumes a higher runoff rate, for example, due to a larger proportion of heavy rainfall events in total precipitation, then the lake levels could have been higher, even with lower total precipitation amounts (see below).
However, there seemed to be another indirect argument in favour of a “pluvial-period” increase in precipitation in the Mediterranean region: According to a widely held believe, dry periods were already “assigned” as times of formation of the widespread CaCO3 crusts. As alternating debris horizons and CaCO3 crusts were generally observed, in the conviction that there was an alternation of dry and wet periods, the CaCO3 crusts were placed into the dry periods, while the formation of the debris-covered slopes was assigned to wetter periods. Today, however, it is clear that the CaCO3 crusts are not surface formations, but Ca-horizons of strongly developed soils, which therefore are climatically associated with formation processes during humid periods (Rohdenburg and Sabelberg, 1969a, b). From this aspect, the way would be clear to regard the morphodynamically active periods as relatively dry periods.
If, on the one hand, all alleged wet-phase-indicators have shown to be unconvincing, there are, on the other hand, now positive indications for arid phases during glacial times. Among these are, firstly, loess deposits in the Mediterranean region (Virgili and Zamarreño, 1957; Riba, 1957; Alia Medina and Riba, 1957; Rohdenburg and Sabelberg, 1969a, b; Brunnacker, 1969; Brunnacker and Lozek, 1969), and secondly, the surprising result of pollen analyses carried out in recent years from central Italy (Bonatti, 1962), southern Spain (Menéndez Amor and Florschütz, 1964) and Greece (van der Hammen et al., 1965), which indicate that during the last glacial period there were open vegetation assemblages and at times even completely forest-free areas in the Mediterranean region, as impressively compiled by Beug (1968).
Based on this, there is no longer a fundamental difference between the tropics and subtropics regarding the palaeoclimatic ideas of phases with pronounced slope erosion. In both zones, climatic conditions must have prevailed that prevented a closed vegetation cover, but rather led to relatively open vegetation types with little protection of soil from erosion. In contrast to the older view, according to which vegetation did not have a significant influence on erosion, the current tendency is to assume a very close dependence between vegetation and geomorphology and to judge climatic changes primarily based on the extent of vegetation changes. But even this view cannot be fully correct, as shown particularly well by the conditions in the extreme drylands.
According to the explanations presented above, one could expect that geomorphological activity increases with enhanced aridity and its corresponding decrease in vegetation density, e.g., both from the north and from the south towards the Sahara. Indeed, this is the case to a certain extent, since the width of the valley floors also increase in this direction and thus, a relatively large part of the relief at the foot of cuestas (“Schichtstufen”) is affected by ongoing formation processes. But it is precisely for these areas that the pluvial hypothesis is still widely used today (cf. Mensching, 1964b), – and this not without merit. On the northern and southern fringes of the Sahara as well as in the central Sahara (cf. Rognon, 1967; Butzer and Hansen, 1968), it is evident that no pedimentation occurs here today, as was the case in this and in wetter areas in the past. This is also well demonstrated by Hövermann's (1967) and Hagedorn's (1967) observations from the Tibesti area, where today, in contrast to earlier conditions, inselbergs and plains are not forming together, but are often separated from each other by peripheral depressions (“Randfurchen”).
In this context, measurements of suspended sediment loads in rivers carried out by Herrmann (1970) in the area of the Sierra Nevada de Santa Marta (Colombia), which is extraordinarily differentiated in terms of climate, are very informative. As was to be expected, the suspended sediment load increases strongly with decreasing precipitation totals due to reduced soil protection, but decreases from its maximum in the zone of dry bare lowland forest, since the effects of heavy rainfall events (expressed as the product of intensity and duration) in areas of the more open vegetation types is lower than in the more precipitation-prone areas. But even within the maximum zone of present-day erosion, the erosion effect is currently very low (today almost exclusively delivering suspended sediment) compared to ancient phases of strong morphodynamic activity with considerable coarse material erosion both on slopes and in rivers (Bartels, 1970). A summary of the erosion measurements of the United States Geological Survey by Langbein and Schumm (1958) also shows a pronounced maximum, which according to Schumm (1965) is located where closed grass covers can no longer form (Fig. 1). Schumm (1965) uses this diagram to reconstruct hydrological conditions during the last glacial period for different areas of the USA based on assumed values of Pleistocene temperature and precipitation changes. This purely actualistic palaeohydrological attempt is, however, doomed to fail, because the conditions favouring erosion during the Pleistocene do no longer exist in the USA (characterised not only by suspended but also by a strong discharge of coarse material both on slopes and in rivers) and therefore cannot be included in the diagram reproduced here as Fig. 1.
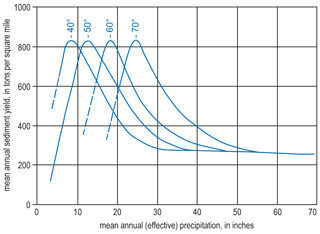
Figure 1Observations of modern erosion as a function of precipitation and temperature in the USA (from Schumm, 1965: Fig. 2).
It is thus evident everywhere that today's vegetation within the wetter areas is too dense for strong erosion, while in the dry areas by contrast, with their very limited soil protection, there is at present not enough morphodynamically effective precipitation. In view of this problem, namely that a simple shift of today's climatic zones is in no way sufficient to explain the ancient phases of strong morphodynamics, climatic conditions must therefore be assumed which do no longer exist between Central Europe and the Gulf of Guinea, between the humid forest and the dry regions in northern South America, and between the humid and the arid regions of the USA3. Since it also appears that strong geomorphological activity prevailed across very large areas at the same time, one may not only expect a uniform climate type with high potential of erosion, but also a considerable range of variation.
If these preconditions are taken into account, then the change compared to the previous phase of limited erosion can only result from a change in factors which simultaneously increases surface runoff and damages the vegetation cover. Both conditions would be fulfilled, for example, by a temperature decrease, if the later is strong enough so that a periglacial environment develops. This easily understandable scenario does not apply to areas outside the periglacial zones during the glacial periods, where simple factor changes (temperature decrease, precipitation decrease, precipitation increase) do not satisfy the two conditions mentioned above. Rather, outside the ancient periglacial areas, only a less even distribution of precipitation can both impair the efficiency and thus the soil-protecting effect of the vegetation and thus promote surface runoff4. This is to be explained in more detail on the basis of the ecological conditions with an accentuated emphasis on the distribution of precipitation, but only pertaining to the currently vegetation-stabilised areas (above 100–150 mm): Under a scenario of weak single rain events and an approximately even distribution of the annual precipitation, as is characteristic for humid areas today, almost all of the precipitation reaching the soil surface (precipitation minus interception) will penetrate the soil and either be absorbed there by the vegetation or enter the groundwater system. With this precipitation distribution, the vegetation is optimally supplied with water, while only a fraction of the precipitation runs off as surface water (e.g., in the middle latitudes during snowmelt), and can hardly cause any erosion due to the dense vegetation. This precipitation distribution is at the same time optimal for strong mineral weathering and further chemical transformations near the surface (soil formation) and at greater depths (saprolite formation).
The heavier the individual rain events and the more irregular the distribution of the annual precipitation, or the more the amounts of precipitation fluctuate from year to year, the less favourable is the water supply for the vegetation. Firstly, a larger proportion of the precipitation water is lost to the vegetation, because not all of it seeps in during heavy rainfall, but some of it runs off surficially. Secondly, the efficiency of the vegetation is greatly reduced by longer dry periods; although the vegetation is drought-adapted, i.e., it can survive dry periods, but its assimilation potential is greatly reduced. Moreover, in xerophytic plants a smaller proportion of the assimilated moisture can be used to increase leaf mass and thus enhance assimilation compared to mesophytic plants. Even small changes in the sense of an uneven increase in precipitation can cause relatively large effects, because the weakening of the vegetation's performance means poorer soil protection against precipitation, more surface water but less percolating water and consequently, in the sense of a self-reinforcing process, a poorer water supply for the vegetation. At the same time, however, the increase in surface runoff increases the erosion effect. However, stronger erosion can in turn reduce the effectiveness of the vegetation. Firstly, direct effects such as partial flushing out of the root system or removal of smaller plants are very important for soil protection. Secondly, flushing reduces the nutrient supply, because in many vegetation types a very large part of the readily available nutrient stocks of the ecosystem is contained within the organic layer and in the often thin Ah-horizon at the surface, so that even moderate erosion can lead to relatively high nutrient losses. The resulting weakening of the effectiveness of the vegetation can, in a second self-reinforcing process, increase surface runoff, erosion, and vegetation damage.
In the case of a climatic change from a uniform to an increasingly uneven distribution of the rainfall, the two self-reinforcing processes described above will likely cause the ecological system to “tip over” from a state where a very high proportion of water percolates into the soil, and effective soil protection by its vegetation cover leading to low erosion, to a second state with sparse vegetation, lower proportions of percolating water and thus increased erosion. In the following, the first state will be referred to as a state of morphodynamic stability, while the second basic state is characterised by morphodynamic activity. Stable periods are characterised by dense vegetation and low erosion, but also by soil formation, whereas these possibilities are much diminished or even non-existent during morphodynamically active periods. Since a vegetation cover is not completely absent in active periods, however, pedogenetic processes will also take place, although, due to their slow progress, they cannot develop in the sense of distinct soil formation if, at the same time, rapid material redistribution takes place due to surface erosion. However, saprolite formation (deep weathering), which is not bound to surface proximity, may also continue in the activity phases. Soil formation originating from the soil surface, however, can only take place in an ecosystem characterised by surface stability. In accumulation sites, well-developed soils intercalated in sediments are therefore the best indicators of phases of ancient stability.
A contradiction to this view could be seen in the fact that today's vegetation is so successfully adapted to very different types of precipitation distribution that, outside the deserts, sufficient soil protection for soil development and morphodynamic stability is guaranteed everywhere. However, this adaptation has only been “provoked” by climate development. Certainly, all sections of the climatic cycles have had an impact on the development of vegetation, but presumably optimal adaptation only took place for the sections with the greatest efficiency of the vegetation, i.e., for those with the most even distribution of precipitation specific to the area and thus the most favourable water supply. The reduced or lack of adaptation of vegetation in a given area to the climate cycle sections with poorer soil water supply could not be fully compensated for by the shifts in vegetation zones associated with climate variability. Not all climate factors were changed to the same extent: for example, the radiation dominated climate and the local wind field, which is important for precipitation formation, have location-specific parameters due to the unchanged positional relationships. It is well known that the Pleistocene periglacial climate of Central Europe cannot be completely equated with any periglacial climate of the present day, as can be seen from the fact that there is no periglacial area today with a strong loess drift that is not restricted to the vicinity of rivers. The threshold value of the “tipping over” from the stable state into the active state will therefore be extraordinarily different from climate area to climate area. Even within these areas there are differences depending on the soil composition5 and the relief. As a first approximation, it can be expected that the general circulation of the atmosphere in the active periods did not differ completely from that of the stable periods, so that the areas with the highest precipitation in the stable periods also received relatively high precipitation in the active periods. The greater the precipitation density, the denser the vegetation can still be at the time when noticeable erosion (partial activity) begins in the transition area between the stability and activity periods. In the tropics, for example, the vegetation will also have been denser in the sections with full activity compared to the dry areas during both the activity and stability periods. I see clear evidence for this view in the fact that in the savanna zone of West Africa, in which the performance of the stable-period vegetation is now anthropo-zoogenically inhibited (fire, grazing), considerable formational activity can already occur in places, even if the water collection area still has a fairly dense vegetation cover (Rohdenburg, 1969). This finding makes it much easier to understand the strong formational activity in areas with high vegetation productivity, because the extent of the climatic change between the stability and activity periods can be estimated as smaller than if one had to assume that present-day desert or at least semi-desert conditions prevailed in the rainforest areas during the activity periods.
The precipitation distribution, whose importance for vegetation in areas without pronounced temperature minima has been generally recognised since the work of Lauer (1952), also represents, according to the view presented here, the most important climatic factor for controlling the ratio of vegetation to erosion. As for the vegetation type, the absolute amount of precipitation is only secondarily important for the vegetation-erosion ratio. Thus, the transition from stability to activity could also occur if the amount of precipitation remains constant. However, it is less likely that the amount of precipitation will remain unaffected by a change in precipitation distribution. Therefore, the two possibilities of increasing activity with (a) increasing and (b) decreasing precipitation quantity come into question.
Case (a) is realised if the more uneven distribution of precipitation consists of an increase in the number of heavy rain events while the number of low and medium-intensity events remains roughly constant. For example, Leopold (1951) correlates increased gully formation at the turn of the last century in New Mexico with a period of increased summer thunderstorms (cf. also Leopold et al., 1963). This case is probably of greatest importance where the vegetation is not very dense, even with morphodynamic stability, and where therefore the heavy rains cannot increase the efficiency of the vegetation, or only to an insignificant extent, i.e., in the distinctly dry areas.
The other possibility of a more accentuated precipitation distribution consists in a shortening of the rainy season or an increase in the number of dry years, which is usually accompanied by a reduction in the amount of precipitation: case (b). However, a reduction in the rainy season only increases erosion if the amount of precipitation decreases less than does the number of humid months, i.e., if the intensity of the individual rains increases relative to the vegetation density. Otherwise, there would only be a simple shift of the existing vegetation zones without a morphodynamic effect. Case (b) thus results in a vegetation zone shift to the drier side compared with case (a), so that for our purposes it is therefore not of primary importance whether precipitation increases or decreases overall.
In Fig. 2, an attempt has been made to show the basic changes in the most important water balance variables over the course of a climate cycle with initially increasing and then decreasing accentuation of the precipitation distribution. Case (b), described above, is implemented with lower total precipitation in the active period than in the stable period. Although for hydrological purposes surface runoff and groundwater runoff are generally combined, our approach requires a separation of the surface runoff. Evapotranspiration (evaporation of soil and plants) and groundwater runoff can be combined into one superordinate unit because of their approximately similar changes in the course of a climate cycle. The most important characteristic of the climatic cycle postulated here in ecological-morphodynamic terms is undoubtedly the opposite direction of the areas of surface runoff on the one hand and evapotranspiration and groundwater runoff on the other. For the drawing of the diagram, it is assumed that in the transitional area between stability and activity (or vice versa) the surface runoff (cross-hatched area) increases much more rapidly than the amount of precipitation decreases, so that the sections with partial activity are relatively short. In addition, a pronounced asymmetry of the “climate wave” was assumed for reasons that will be discussed below. For the surface runoff in the stability periods, a mediating value was entered between almost zero, with dense vegetation and very uniform precipitation distribution, and higher values both in the dry areas and in the humid tropics with a dense vegetation cover due to the high proportion of heavy rainfall in both areas.
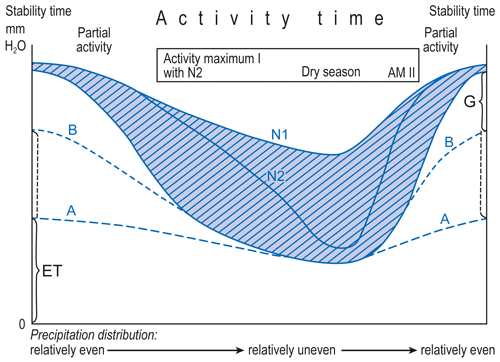
Figure 2Surface runoff (cross-hatched), groundwater runoff (G) and evapotranspiration (ET) over the course of a climate cycle with initially increasing and later decreasing accentuation of the annual precipitation distribution. N = precipitation amount; A, B = explanation in text.
This condition with very high erosion of suspended sediment on rather flat slopes should be considered as a state of stability, contrary to other opinions (e.g. Büdel, 1963, 1970, despite abundant evidence to the contrary and without any discussion of the problem). Conditions of stability could be deduced, because on the one hand soil formation is taking place there today, and on the other hand the former periods of activity were characterized by coarse material transport on all slopes, even in the humid and wet-dry humid tropics (Fölster, 1964, 1969; Rohdenburg, 1969). This view also corresponds, for example, with the fact that today in the humid tropics on rocks of high infiltration capacity (such as the coarse-grained Falsebedded Sandstone of the Upper Cretaceous in SE Nigeria) there is no surface runoff at all on slopes below 5∘, whereas pedimentation occurred there during the last period of activity due to high surface runoff (Herrmann, 1968; Rohdenburg, 1969, 1970)!
Just as the surface runoff of the stability periods varies extraordinarily strongly depending on climate, rock and relief, so do the differences in the relationship between groundwater runoff and evapotranspiration. This range of variation is to be taken into account by drawing the two curves A and B. The curves A and B apply to areas with a high stability time. Curve A applies to areas with very high precipitation, in which the surplus of soil water reaching the groundwater is large despite high water consumption by the vegetation. With decreasing precipitation, evapotranspiration decreases, but the surplus of soil water not consumed by vegetation decreases even more, so that groundwater runoff can be quite low in a permanently humid midlatitude climate (e.g., Central Europe) (e.g., curve B), but decreases to zero in drylands.
Since the surface runoff is relatively small everywhere under today's conditions of predominant morphodynamic stability, the total runoff also changes in the same direction as the amount of precipitation. One is therefore inclined to interpret higher lake levels in the past as times of higher precipitation. However, this simple relationship can only be valid for stability conditions, but not unrestrictedly for activity conditions with their considerably increased surface runoff, as can also be seen from Fig. 2. In all normally humid to semi-arid areas (groundwater discharge according to curve B or lower) it is to be expected that during the transition to the activity period the decrease in groundwater discharge will be overcompensated by the increase in surface runoff, so that the consequence is an increased total runoff. Lake level highs, which are linked to an activity period in these areas, are therefore not likely to be due to increased groundwater yield as a result of higher precipitation, but to higher surface runoff as a result of more accentuated precipitation distribution, presumably even under reduced total precipitation. Two climate areas, however, do not fall within the scope of this important relationship:
In areas with very high stability-time groundwater discharge (e.g., curve A) due to very high precipitation, the decrease in groundwater discharge is not likely to be completely compensated by the increase in surface runoff in the transition to activity conditions. However, this case is of little importance for the question of ancient lake highstands, since the stability-time precipitation-rich areas are characteristically poor in endorheic basins.
Extremely arid areas show only low morphodynamic activity despite a lack of vegetation due to insufficient precipitation (see above). Both periods of ancient soil formation and periods of ancient strong morphodynamic activity, as found in some of these areas (e.g., in Nubia by Butzer and Hansen, 1968), can only be caused by former higher precipitation, which will also have caused higher runoff. However, it can be assumed that the total runoff was greater during the activity periods than during the soil formation periods, unless these were characterised by very high humidity. Any attempt to analyse higher lake levels in arid areas therefore first requires an investigation into whether they fall either in a period of increased morphodynamic activity or in a period of soil formation.
The previous considerations assumed that the maximum of precipitation reduction coincides with the maximum of morphodynamic activity. This assumption may be true for certain areas or for certain periods of activity (see below), but according to many field observations it is certainly not true everywhere or for all periods of activity. In the case of a very pronounced reduction in precipitation with increasing accentuation of the precipitation distribution, as shown in Fig. 2 by the curve N2, the surface runoff initially increases rapidly after vegetation stabilisation has been overcome. However, it then decreases as the amount of precipitation continues to decrease. When precipitation increases again, we must expect a reversal of this development, i.e., a renewed activity maximum before stabilisation of the surface through vegetation. The activity period is then divided into three parts.
Between two activity maxima, a dry period is interposed, which can be characterised by strong aeolian activity in addition to the lower erosion intensity. A larger number of field observations from the subtropics indicate that the activity periods were not symmetrical, but rather highly asymmetrical, as shown in Fig. 2. The dry season is shifted towards the end of the climate cycle and the activity maximum II is clearly weaker (because it is shorter) than the activity maximum I; in extreme cases, the activity maximum II can almost completely disappear due to a very high degree of asymmetry6.
As far as the last glacial period in the Mediterranean area is concerned, Butzer (1958) and Messerli (1967) also advocate a very early maximum of the erosion effect. Similar conditions are also present in Jordan, where the detailed investigations by Huckriede and Wiesemann (1968) inspire a comparison. According to these authors, the most recent large lake highs in Jordan coincide with the Early and Middle Würm glaciation (Lake Lisan, “Pluvial Lake” of El Jafr). This was followed by a very pronounced dry period: (“post-Lisan dry period”) with strong aeolian activity (abrasion, deflation, dunes) and subsequently, still in the Late Würm, renewed stronger fluvial activity with formation of the El Jafr sebkha, incision of wadis into the “pluvial limestones” and deposition of gravels and clays in other wadi systems. In the present, within the rain pan “only minor redeposition occurs in the uppermost layers during winter rains” (Huckriede and Wiesemann, 1968, pp. 82–83). The obvious similarity of this climatic sequence, also with regard to the asymmetry of the climatic wave, presupposes, however, a coincidence of lake highs and strong slope erosion (see above). Reinhold Huckriede (personal communication, 1970), with regard to the formation of Lake Lisan, and in agreement with this derivation, considers pronounced heavy winter rains. For the second activity maximum, Huckriede and Wiesemann (1968) also report increased wadi activity. An apparent deviation is that the Holocene is also considered arid and is characterised by dune formation, whereas according to Fig. 2 relatively humid conditions should prevail during the Holocene stability period. This discrepancy is due to the fact that the main catchment area of the El Jafr basin lies in areas with higher precipitation, and the diagram therefore applies mainly to these, and less to the basin itself.
In Central Europe, the climate wave of the cold-warm cycles is clearly superimposed by secondary and tertiary oscillations, which leads to a division of the glacial periods into stadials and interstadials. The same phenomenon can also be observed outside the former periglacial areas. Investigations of thick slope and alluvial fan sediments on the Balearic Islands and in Morocco revealed a subdivision through fossil soils as rich as, for example, in Central European loess profiles. From this it must be concluded that the secondary oscillations, like the primary oscillations, have an effect not only thermally but also on the precipitation distribution and led to (albeit in part only short-term) states of stability within the glacial periods. Since in the subtropics and tropics it is not so much different temperatures but rather much greater differences in moisture content that determine the typological and intensity-related formation of fossil soils, in contrast to Central Europe, it is not possible to determine whether they originate from an interglacial period or whether they were formed as a result of a secondary oscillation within a glacial period.
Without a detailed stratigraphy subdividing the primary oscillations, which exists only in rudimentary form in the subtropics and tropics, it is not possible to determine with certainty whether the Pleistocene climate fluctuations had similar effects in large areas of the Earth. Accordingly, Monod (1964), in a summary essay on “The Late Tertiary and Pleistocene in the Sahara”, puts Wayland's (1933)7 statement “I fear you are on rather ticklish ground with the Pluvials. We have yet much to learn ... we need more facts” and writes in the text (p. 119): “The literature, however considerable, deals more with theories, opinions, and suppositions than with observed facts. These themselves are often subject to interpretations which are hazardous, ambivalent, or even impossible...” (cf. also Frenzel, 1967, p. 183). In this sense, this paper was not so much intended as a new “pluvial theory”, but rather as a contribution to the palaeoclimatic interpretation of “observed facts”, namely that it is essential to fundamentally separate soil formation and morphodynamic activity from each other and not to subsume them under the same term (“pluvial”).
This distinction could also invalidate the widespread idea of a temporal alternation of “pluvials” in the regions north and south of the Sahara (e.g., Balout, 1952; Tricart, 1956; Büdel, 1963). North of the Sahara, evidence of morphodynamic activity and in the humid tropics, evidence of soil formation, have been used as “pluvial” indicators. Based on the distinction between stability and activity periods, however, one tends to arrive at the opposite idea of far-reaching parallels between the subtropics and the tropics, because in both areas stability conditions with soil formation prevail today, while the last glacial period in both zones was (at least temporarily) characterised by morphodynamic activity and a pronounced dry period (Hermann Flohn, personal communication, 1970). However, the extent to which the activity maxima or activity phases separated by secondary oscillations with soil formation are of the same age over a wide area cannot be estimated with certainty at present. Even if the activity periods are largely simultaneous, the activity maxima of different areas do not have to be strictly simultaneous, even according to the conclusions drawn from Fig. 2. For example, in areas with high precipitation and strong vegetation, the activity period could begin relatively late and reach its maximum during the strongest reduction in precipitation, while in drier areas partial activity and activity occur early, but during the maximum reduction in precipitation a dry period separates two activity maxima from each other (curve N2).
Moreover, the previous considerations apply more or less only to those areas where the surface would currently be stabilised (> 100–150 mm precipitation) with anthropo-zoogenically undisturbed vegetation. In the areas with lower precipitation today (semi-desert and full desert areas), both earlier soil formation and activity periods must have experienced higher precipitation than today (see above). The present morphodynamic stability of the arid regions also suggests that the formation periods of the paleosoils took place in worldwide stability periods with considerably greater humidity than today8, in which the desert area of the Sahara was thus constricted both from the north and from the south. A positive indication of this can be seen, for example, in the fact that the postglacial climatic optimum was wetter than today both in the mid-latitudes (Frenzel, 1967) and north and south of the Sahara (Butzer, 1958). Conversely, during the glacial period dry phases, the Sahara expanded on both sides. However, the activity periods of the arid regions remain problematic, which can only be explained by simultaneous accentuation of the precipitation distribution and increased precipitation. If such conditions, which have not been included in Fig. 2, prevailed in larger areas, it must be expected that morphodynamic activity also spread to semi-arid areas, but that vegetation stabilisation could not be overcome in more humid areas. This is followed by an alternation of activity maxima between the dry areas on the one hand and the wet areas on the other9, in both the subtropics and the tropics.
The lack of “facts”, essentially due to the absence of a detailed stratigraphy, does not allow any decisions and at the same time prohibits the continuation of these hypothetical discussions. However, it seemed essential to me to indicate which consequences must be drawn from a reconsideration of the ambiguous pluvial concept and in which direction future research results can be expected.
This paper was edited by Frank Preusser.
Alia Medina, M. and Riba, O.: Livret – Guide de l'excursion C4: Manzanares et Tolede, INQUA V. Congr. Intern., Madrid-Barcelona, Spain, 1957.
Balout, L.: Pluviaux interglaciaires et Préhistoire saharienne, Trav. Inst. Rech. Sahar., 8, 9–21, 1952.
Bartels, G.: Geomorphologische Höhenstufen der Sierra Nevada de Santa Marta (Kolumbien), Gießener Geogr. Schr., 21, 1970.
Beug, H. J.: Probleme der Vegetationsgeschichte in Südeuropa. Ber. d. Dtsch. Botan. Ges., 80, 682–689, 1968.
Bigarella, J. J.: Slope development in south eastern and southern Brazil, Z. Geomorphol., 10, 150–160, 1966.
Bonatti, E.: Late Quaternary pollen sequences from Central Italy, Pollen et Spores, 4, 335–336, 1962.
Brückner, W.: The mantle rock (“laterite”) of the Gold Coast and its origin, Geol. Rdsch., 43, 307–327, 1955.
Brunnacker, K.: Affleurements de loess dans les regions Nord-Mediterranneennes, Revue de Geographie et de Geologie dynamique, 11, 325–334, 1969.
Brunnacker, K. and Lozek, V.: Löß-Vorkommen in Südostspanien, Z. Geomorphol., 13, 297–316, 1969.
Büdel, J.: Die pliozänen und quartären Pluvialzeiten der Sahara, E&G Quaternary Sci. J., 14, 161–187, https://doi.org/10.3285/eg.14.1.18, 1963.
Büdel, J.: Pedimente, Rumpfflächen und Rückland-Steilhänge, Z. Geomorphol., 14, 1–57, 1970.
Butzer, K.: Quaternary stratigraphy and climate in the Near East, Bonner Geograph. Abh., 24, p. 157, 1958.
Butzer, K.: Paleoclimatic implications of Pleistocene stratigraphy in the mediterranean area, Ann. New York Acad. Sci., 95, 449–456, 1961.
Butzer, K.: Pleistocene geomorphology and stratigraphy of the Costa Brava Region (Catalonia), Akad. Wiss. Lit. Mainz, Abh. Math.-Kat. K., Jg. 1964, Nr. 1, Mainz, Germany, 1964a.
Butzer, K.: Climatic-geomorphologic interpretation of Pleistocene sediments in the Eurafrican Subtropics, in: African Ecology and Human Evolution, edited by: Howell, F. C. and Bourliere, F., London, UK, 1–27, 1964b.
Butzer, K. and Hansen, C. L.: Desert and river in Nubia, University of Wisconsin Press, Madison, USA, 562 pp., 1968.
Coque, R.: La Tunisie présaharienne, Colin, Paris, France, 1962.
De Ploey, J.: Position geomorphologique, genese et chronologie de certains depots superficiels au Congo occidental, Quaternaria, VII, 131–154, 1965.
Fölster, H.: Morphogenese der südsudanesischen Pediplane, Z. Geomorphol., 8, 393–423, 1964.
Fölster, H.: Slope development in SW-Nigeria during Late Pleistocene and Holocene, Gießener Geogr. Schr., 20, 3–56, 1969.
Frenzel, B.: Die Klimaschwankungen des Eiszeitalters, Vieweg, Braunschweig, Germany, 1967.
Hagedorn, H.: Beobachtungen an Inselbergen im westlichen Tibesti-Vorland, Berliner Geogr. Abh., 5, 17–22, 1967.
Haude, W.: Erfordern die Hochstände des Toten Meeres die Annahme von Pluvial-Zeiten während des Pleistozäns?, Meteorol. Rdsch., 22, 29–40, 1969.
Herrmann, R.: Methoden und Ergebnisse einer hydrographischen Landesaufnahme von Ostnigeria, Erdkunde, 22, 89–100, 1968.
Herrmann, R.: Zur regionalhydrologischen Analyse und Gliederung der nordwestlichen Sierra Nevada de Santa Marta (Kolumbien), Gießener Geogr. Schr., 23, 88 pp., 1970.
Hövermann, J.: Hangformen und Hangentwicklung zwischen Syrte und Tschad, L'Evolution des Versants, Les Congres et Colloques de l'Universite de Liege, Liege, Belgium, 40, 139–156, 1967.
Huckriede, R. and Wiesemann, G.: Der jungpleistozäne Pluvial-See von El Jafr und weitere Daten zum Quartär Jordaniens, Geologica et Palaeontologica, 2, 73–95, 1968.
Kukla, J.: Die zyklische Entwicklung und die absolute Datierung der Löß-Serien, Periglazialzone, Löß und Paläolithikum der Tschechoslowakei, Brno, Czech Republic, 75–95, 1969.
Langbein, W. B. and Schumm. S. A.: Yield of sediment in relation to mean annual precipitation, Amer. Geophys. Union Trans., 39, 1076–1084, 1958.
Lauer, W.: Humide und aride Jahreszeiten in Afrika und Südamerika und ihre Beziehungen zu den Vegetationsgürteln, Bonner Geogr. Abh., 9, 15–66, 1952.
Leopold, L. B.: Rainfall frequency, an aspect of climatic variation, Trans. Amer. Geophys. Union, 32, 347–357, 1951.
Leopold, L . B., Leopold, E. B., and Wendorf, F.: Some climatic Indicators in the Period A.D. 1200–1400 in New Mexico, Changes of Climate, Arid Zone Research, UNESCO, Paris, France, 265–280, 1963.
Ložek, V.: Quartärmollusken der Tschechoslowakei, Rospravy Ústredního Ústavu Geologickeho, Praha, Czech Republic, 31 pp., 1964.
Mabesoone, J. M.: Relief of northeastern Brazil and its correlated sediments, Z. Geomorphol., 10, 419–453, 1966.
Menéndez Amor, J. and Florschütz, F.: Results of the preliminary palynological investigation of samples from a 50 m boring in southern Spain, Bol. R. Soc. Española Hist. Nat. (Geol.), 62, 251–255, 1964.
Mensching, H.: Karst und Terra rossa auf Mallorca, Erdkunde, 9, 188–196, 1955.
Mensching, H.: Die regionale und klimatisch-morphologische Differenzierung von Bergfußflächen auf der Iberischen Halbinsel (Ebrobecken – Nordmeseta – Küstenraum Iberiens), Würzburger Geogr. Arb., 12, 141–158, 1964a.
Mensching, H.: Zur Geomorphologie Südtunesiens, Z. Geomorphol., 8, 424–439, 1964b.
Messerli, B.: Die eiszeitliche und die gegenwärtige Vergletscherung im Mittelmeerraum, Geogr. Helv., 22, 105–228, https://doi.org/10.5194/gh-22-105-1967, 1967.
Meyer, B. and Moshrefi, N.: Experimente zur Entstehung von Einwaschungs-Tonlamellen in Böden und Sedimenten durch Fließvorgänge im ungesättigten Feuchtezustand, Masch.-Schr. Mskr., im Druck in Göttinger Bodenkdl. Ber., Göttingen, Germany, 1969.
Monod, T.: The late Tertiary and Pleistocene in the Sahara, in: African Ecology and Human Evolution, edited by: Howell, F. C. and Bourliere, F., London, UK, 117–229, 1964.
Nye, P.: Some soil-forming processes in the humid tropics: I A field study of a catena in the West African forest, J. Soil Sci., 5, 7–21, 1954.
Nye, P.: IV The action of the soil fauna, J. Soil Sci., 6, 73–83, 1955.
Riba, O.: Livret-guide de l'excursion L2: Terrasses du Manzanares et du Jarama aux environs de Madrid, INQUA V. Congr. Intern., Madrid-Barcelona, Spain, 1957.
Rognon, P.: Climatic influences on the African Hoggar during the Quaternary, Based on geomorphologic observations, Ann. Assoc. Amer. Geogr., 57, 115–127, 1967.
Rohdenburg, H.: Hangpedimentation und Klimawechsel als wichtigste Faktoren der Flächen- und Stufenbildung in den wechselfeuchten Tropen an Beispielen aus Westafrika, besonders aus dem Schichtstufenland Südost-Nigerias, Gießener Geogr. Schr., 20, 57–152, 1969.
Rohdenburg, H.: Hangpedimentation und Klimawechsel als wichtigste Faktoren der Flächen- und Stufenbildung in den wechselfeuchten Tropen, Z. Geomorphol., 14, 58–78, 1970.
Rohdenburg, H. and Meyer, B.: Zur Feinstratigraphie und Paläopedologie des Jungpleistozäns nach Untersuchungen an südniedersächsischen und nordhessischen Lößprofilen, Mitt. Dtsch. Bodenkdl. Ges., 5, 1–135, 1966.
Rohdenburg, H. and Sabelberg, U.: “Kalkkrusten” und ihr klimatischer Aussagewert – neue Beobachtungen aus Spanien und Nordafrika, Göttinger Bodenkdl. Ber., 7, 3—26, 1969a.
Rohdenburg, H. and Sabelberg, U.: Zur landschaftsökologisch-bodengeographischen und klimagenetisch-geomorphologischen Stellung des westlichen Mediterrangebietes, Göttinger Bodenkdl. Ber., 7, 27–47, 1969b.
Schumm, S. A.: Quaternary palaohydrology, in: The Quaternary of the United States, edited by: Wright, H. E. and Frey, D. G., Princeton, USA, 783–794, 1965.
Tricart, J.: Tentative de correlation des periodes pluviales africaines et des periodes glacaires, C. R. Somm. Soc. Geol. France, 9–10, 164–167, 1956.
Tricart, J.: Le modele de regions chaudes forets et savanes, Traité de Géomorph., Tome, vol. 5, Paris, France, 1965.
van der Hammen, T., Wijmstra, T. A., and van der Molen, W. H.: Palynological study of a very thick peat section in Greece, and the Würmglacial vegetation in the Mediterranean region, Geologie en Mijnbouw, 44, 37–39, 1965.
Vincent, P. L.: Les formations meubles superficielles au sud du Congo et au Gabon, in: Terrains d'álteration et de recouvrement en zone intertropicale, edited by: Vogt, J. and Vincent, P. L., Bull. du B. R. G. M., 4, 1–111, 1966.
Virgili, C. and Zamarreno, J.: Le Quaternaire continental de la plaine de Barcelone, INQUA V. Congr. Intern. Livretguide de l'excursion B1: Environs de Barcelone et Montserrat, 7–16, Madrid-Barcelona, Spain, 1957.
Vogt, J.: Le complex de la stone-line, Mise au point, Bull. B. R. G. M., 4, 3–51, 1966.
Volk, O. H. and Geyger, E.: “Schaumboden” als Ursache der Vegetationslosigkeit in ariden Gebieten, Z. Geomorphol., 14, 79–95, 1970.
Wiche, K.: Geomorphologische Studien in Südostspanien (Provinz Murcia), Mitt. österr. Geogr. Ges., 101, 390–395, 1959.
Wiche, K.: Beiträge zur Formenentwicklung der Sierren am unteren Segura (Südostspanien), Mitt. d. Österr. Geograph. Ges., 103, 125–157, 1961.
From a pedological point of view, stone pavements and debris horizons were initially regarded as subterranean phenomena caused by termite activity and soil creep (Nye, 1954, 1955). This view is now obsolete, since the subaerial origin of these coarse materials has been sufficiently proven, and it is also clear that no deep soil creep takes place on shallow slopes.
Percolating water often cannot penetrate the soil undifferentiated, but differentiation into a multitude of thin water horizons takes place. If, after a precipitation event, the soil air cannot escape upwards quickly enough in the absence of continuous coarse pores, but instead is trapped by the percolation water front, a percolation water front finally occurs on compressed “air cushions”, i.e., the formation of “water bands” (in the two-dimensional profile image) (Meyer and Moshrefi, 1969) with high to total pore filling by water, which are arranged parallel to the surface. As a result of the growing percolating water load, air cushions break out locally, but without disintegration, although at greater depth, the water front – air cushion formation is repeated. Overall, the percolation water movement is vertically directed, yet within the individual water bands, lateral movement, downslope or to breakthrough points, prevails. According to this differentiation of percolating water, accumulation horizons often have a layered structure, such as iron and humus bands in podsols and clay bands in parabrown soils (“Tüxen bands”). The calcareous lamellar series of CaCO3 crusts represent a completely analogous differentiation of Ca horizons. The air-cushion-water-band theory of Meyer and Moshrefi (1969) can thus explain both the multitude of lamellae formed simultaneously one on top of the other and the lateral movement of water, which has always been emphasised for the formation of the calcareous lamellae and for which until now only a superficial origin seemed to be possible.
It is interesting that Büdel (1963), despite his actualistic conception of ancient morphodynamics, is also forced to adopt a palaeoclimate conception that cannot be interpreted in an actualistic way.
In this context, it is important that Hermann Flohn (personal communication, 1970) concludes for the Pleistocene glacial periods that the convective character of precipitation over the tropical and subtropical continents increased, which should result in an accentuation of the precipitation distribution.
In a recently published very interesting paper Volk and Geyger (1970) consider “foam soils as a cause of the lack of vegetation in arid regions”. Personally, I can only recognise the behaviour of the soil air in dry silt-rich soils, which always influences the penetration of percolating water (cf. footnote 2), as one of many site factors that contribute to the change from stability to activity. Even in the dry areas of the Mediterranean region, the sites that are now free of vegetation would certainly be covered with vegetation if man had not interfered with the landscape balance. As a result of this disturbance, sites with low infiltration capacity have naturally been affected the most, so that they first show full activity. On the other hand, at the end of a period of activity, such sites will hinder vegetation regrowth for the longest time, so that they can also remain completely vegetation-free in extreme dry areas (cf. the examples from southern Morocco). Volk and Geyger are to be thanked for pointing out the importance of soil air for the water balance, which should not be underestimated (cf. footnote 2 and Rohdenburg and Meyer, 1966) and have worked out such a simple indicator for soils with low infiltration capacity.
This asymmetry has its parallel in Central Europe, where it has been known for a long time that the greatest cold and dryness within the last glacial period is only characteristic of the Late Würm glaciation (cf. Ložek, 1964). According to Kukla (1969), who assumes a similar climatic structure for all glacial cycles, this also applies to all other glacial periods. Kukla (1969) justifies the asymmetry of the climate wave with the fact that the build-up of the inland ice masses lags considerably behind the triggering process of climate change, and that therefore all subsequent phenomena (strongest cooling due to greater albedo, strongest drought due to lowest evaporation with strongest cooling) are also shifted towards the end of a glacial cycle. Hermann Flohn (personal communication, 1970) also deduces from a purely geophysical model that in every glacial period the glacial maximum and perhaps also the Late Glacial are characterised by a markedly arid phase of worldwide distribution.
Translators' note: This citation has no entry in the list of references as per original article.
Perhaps in stability periods, based on secondary oscillations with temperature-related low evaporation and thus higher humidity (cf. Frenzel, 1967)?
Although not identical, in some respects similar ideas were developed by Wolfgang Andres (colloquium lecture Geograph. Inst. Frankfurt, April 1970, on southern Morocco).