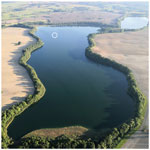
Lakes and trees as climate and environment archives: the TERENO Northeastern German Lowland Observatory
Achim Brauer
Ingo Heinrich
Markus J. Schwab
Birgit Plessen
Brian Brademann
Matthias Köppl
Sylvia Pinkerneil
Daniel Balanzategui
Gerhard Helle
Theresa Blume
Brauer, A., Heinrich, I., Schwab, M. J., Plessen, B., Brademann, B., Köppl, M., Pinkerneil, S., Balanzategui, D., Helle, G., and Blume, T.: Lakes and trees as climate and environment archives: the TERENO Northeastern German Lowland Observatory, DEUQUA Spec. Pub., 4, 41–58, https://doi.org/10.5194/deuquasp-4-41-2022, 2022.
Robust reconstruction of past climate and environmental change based on proxy data obtained from natural archives requires an in-depth understanding of the processes and mechanisms that form and determine these proxies. Here we present comprehensive long-term monitoring projects for seasonally laminated (varved) lake sediments and tree rings in the northern German lowlands. The two monitoring sites are located in the nature park Nossentiner/Schwinzer Heide (Tiefer See) and in the Müritz National Park (tree rings) and are an integral part of the Helmholtz TERrestrial ENvironmental Observatories (TERENO) infrastructure initiative. Both sites are located in the close vicinity of moraine deposits of the main ice advance of the Pomeranian phase of the Weichselian glaciation. This field guide provides an introduction to the local morphologies and landscapes as well as details of the monitoring concepts and some selected results.
- Article
(23669 KB) - Full-text XML
- BibTeX
- EndNote
Climate and environmental changes in the past are reconstructed from a wide range of chemical, physical and biological proxy data obtained from different types of geoarchives. For a robust interpretation of these proxy data it is crucial to understand how climate and environmental signals translate into proxy data. A common approach for an advanced reading of proxy data is to monitor processes by observing and measuring lacustrine sedimentation and growth rates of trees in combination with meteorological parameters at high temporal resolution. This enables us to identify which weather parameters are well reflected and which are less well reflected in sediment records or tree rings. It can be assumed that climate information is different in different geoarchives, and, therefore, the largest gain is achieved by combining several archive types. Here, we focus on annually laminated lake sediments and tree rings as two seasonally resolving archives. A critical factor for monitoring approaches is their duration, which often is limited due to the high cost and human resources required. However, given the pronounced interannual variability and the need to observe effects especially of rare extreme years and events, more information and crucial process understanding will be gained the longer an observation can be maintained. The monitoring sites presented here have run for a decade as an integrated part of the TERrestrial ENvironmental Observatories (TERENO) initiative.
1.1 TERENO
Global change has triggered a number of environmental changes, such as alterations in climate, land productivity, water resources, atmospheric chemistry and ecological systems. Finding solutions to the impact of global change is one of the most important challenges of the 21st century. Therefore, the Helmholtz Association has set up four terrestrial observatories, forming the focus regions of the TERENO network. TERENO stands for TERrestrial ENvironmental Observatories (Zacharias et al., 2011). The observatories were selected to be representative of Germany and other central European regions with the highest vulnerability with respect to climate change effects (Zebisch et al., 2005).
The overall aim of TERENO is to monitor climate change impacts on the terrestrial system in Germany for at least 15 years. Terrestrial systems in TERENO comprise the subsurface environment, the land surface including the biosphere, the lower atmosphere and the anthroposphere. These systems are organized along a hierarchy of spatial scales ranging from the local scale to the regional scale. Furthermore, temporal scales ranging from directly observable monitoring periods to longer periods of up to millennial timescales derived from bio- and geoarchives are considered. With regard to the latter, TERENO concentrates on precisely dated and annually to sub-seasonally resolved synchronized long-term data from lake sediments and tree rings. The overarching goal is to develop process studies based on comprehensive monitoring of bio- and geoarchives to gain a robust understanding of the climate and environmental signal transfers into these archives (Zacharias et al., 2011).
1.2 Monitoring aims
In addition to monitoring the regional impacts of recent climate change, the specific goal of the Northeast German Lowland Observatory (TERENO-NE) is to extend climate and environmental time series several millennia back in time by seasonally resolving proxy data from lake sediments (varves) and tree rings. Such time series are essential to evaluate ongoing change with respect to natural variability. This approach requires in-depth understanding of geochemical, biological and sedimentological (in the case of lakes) proxies for an advanced and robust interpretation. Our approach, therefore, includes the integration of analyses of sediment profile and tree-ring series with observations of changes and their causal mechanisms through high-resolution monitoring. In doing so, weather and climate parameters as well as site-specific responses that control the formation of the proxies can be deciphered (Heinrich et al., 2018).
1.3 Site selection
In an ideal world, the lake and tree-ring observatories should be at exactly the same location, but due to the specific requirements for each observatory, we had to select two different sites locations within the northeastern German lowlands. After detailed site surveys, we selected Tiefer See in the nature park Nossentiner/Schwinzer Heide for lake monitoring because in this lake presently annual laminations (varves) are formed which are required for seasonal reconstructions from sediment records. The tree-ring observatory was installed in a southeastern direction from Tiefer See in the catchment of Hinnensee in the Müritz National Park because at this location we found a forest with old trees and various tree species growing along a pronounced altitudinal gradient with respect to the shore of Hinnensee. This gradient is required to fully assess the various responses of different trees and tree species growing under different groundwater conditions. Since the distance between the lake and tree monitoring sites is less than ca. 55 km, the climatic conditions are considered largely identical. The same holds for the geological and geomorphological situation because both sites are located in the close vicinity of the main W2 ice advance during the Pomeranian stage of the last glaciation (Fig. 1).
1.4 Present-day climate
The climate is warm–temperate at the transition from oceanic to continental conditions (Scharnweber et al., 2011). The average monthly temperatures vary between 0 ∘C in January to 17–18 ∘C in July with maxima of up to 30 ∘C and minima down to −5 ∘C. The mean annual precipitation amounts to 560–570 mm with seasonal averages ranging between ca. 40 mm during winter months and ca. 60 mm in summer months (Heinrich et al., 2019).
Tiefer See has been selected for long-term monitoring mainly because it presently forms annual laminations, i.e. calcite varves. Another advantage of this lake is the lack of any infrastructure on the lake shore like roads and buildings. On the one hand, this minimizes direct human impact on the lake, but, on the other hand, it complicates the logistics for maintaining the monitoring especially during wet seasons when access to the lake is challenging.
Tiefer See is located at the eastern margin of the nature park Nossentiner/Schwinzer Heide at 53∘35.5′ N, 12∘31.8′ E and is part of the Klocksin lake chain, a subglacial gully system formed during the last glaciation. Abundant erratics on the shoreline of the lake indicate its close proximity to the W2 terminal moraines of the Pomeranian phase. The Pomeranian ice advance has been radiocarbon dated at ∼16.5 ka cal BP (e.g. Uścinowicz, 1999) and at about 20 ka by optically stimulated luminescence (OSL) dating (Lüthgens et al., 2011) and recalibrated exposure dating (Hardt and Böse, 2018). The catchment is mainly formed by glacial tills except a small shallow part in the SE which consists of old lake deposits that were accumulated when there was a higher lake level, presumably during the late glacial period.
The Klocksin lake chain begins north of the former ice margin and extends for ca. 16 km from NNE to SSW. It includes the four lakes Flacher See (64.4 m a.s.l.), Tiefer See, Hofsee (62.7 m a.s.l.) and Bergsee (62.6 m a.s.l.). Today, Tiefer See is connected to Hofsee in the south, while the connection to Flacher See in the north has been channelized in a tunnel after construction of a railway dam between the two lakes in the late 19th century. The surface area of Tiefer See is ca. 0.75 km2, and the catchment area is about 5.5 km2. With a maximum depth of 62–63 m Tiefer See is the deepest lake of the lake chain. It has no major inflow and outflow. The present-day lake water is mesotrophic, and the circulation mode is either mono- or dimictic, depending on the formation of a winter ice cover (Kienel et al., 2013). On the shoreline of the lake a narrow band of predominantly large oak trees forms a kind of shelter separating the lake from intensive agriculture with crop fields in the catchment (Fig. 2).
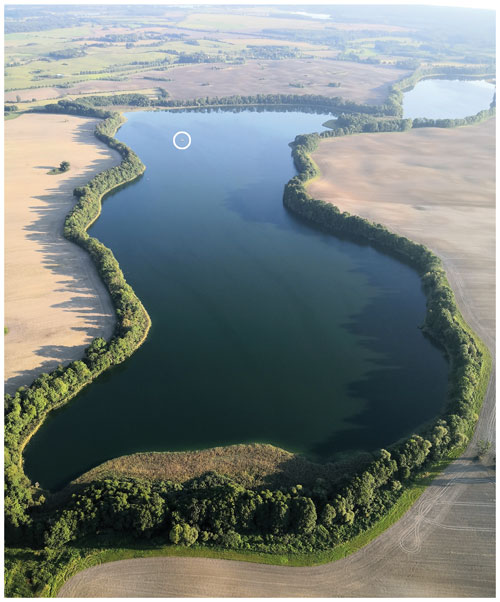
Figure 2View of Tiefer See (53∘35.5′ N, 12∘31.8′ E) from the north. White circle shows position of the monitoring station on a platform anchored at the deepest part of the lake basin.
Tiefer See is one of the few lakes in northern Germany that has formed varves for about 100 years. During other time intervals in the past, for example, the Medieval Warm Period, varves have also formed, which, however, differ in structure and composition from the modern varves (Dräger et al., 2017), The alternation between varved and non-varved sediment sections throughout the Holocene depicts the high sensitivity of this lake with respect to varve formation and preservation. This characteristic makes the lake an ideal site to investigate processes that cause the presence of varves in order to ultimately use these varves as proxies for climate and environment changes in the past. So far, reports on climatic and human factors influencing sedimentation (e.g. Kienel et al., 2013, 2017; Dräger et al., 2017; Theuerkauf et al., 2015; Nantke et al., 2021) have emphasized the difficulty in distinguishing between both factors from analyses of the sediment record. This is exemplarily demonstrated in a detailed reconstruction of Holocene lake level changes proving that lake levels are not solely controlled by climate but by a complex interaction between climate, vegetation and human impact (Theuerkauf et al., 2022). The latter can be either indirect through modification of vegetation cover, direct through drainage or damming measures, or both. Interestingly, decadal to centennial lake level fluctuations are superimposed by a generally increasing trend from the early Holocene until today, which probably is related to changes in the Earth's orbit resulting in increasing winter insolation and decreasing summer insolation and thus reduced seasonal amplitudes. Presumably, these insolation changes caused a modification of atmospheric circulation with an increase in moist air masses from the Atlantic reaching northeastern Germany. Comparing long- and short-term lake level fluctuations shows that the total amplitude of >9 m by far exceeds observed variations of ca. 1 m in the last 2 decades.
2.1 The sediment record
Seven long and overlapping sediment core sequences have been obtained from the deepest part of the lake basin with the GFZ UWITEC piston coring device during three expeditions in 2011, 2013 and 2019. A composite profile from cores taken in 2011 and 2013 (TSK11-A, TSK11-B and TSK11-C and TSK13-E and TSK13-F) has been established based on robust macroscopic and microscopic correlation layers (Fig. 3; Dräger et al., 2017). Two minor gaps due to coring issues below 750 cm have been bridged with two additional core sequences from 2019 (TSK19-H, TSK19-K). This final and continuous composite profile is about 1200 cm long and reaches glacial sands and gravel at the base, which were deposited after melting of dead ice. Lacustrine sedimentation commenced in the late Allerød or early Younger Dryas. The sharp onset of organic sediments at 1138 cm sediment depth coincides with the pollen-defined onset of the Holocene (Martin Theuerkauf, personal communication, 2021). Holocene sediments mainly consist of three components, authigenic and terrestrial organics, biogenically formed calcite, and diatom frustules. Due to the lack of a major inflow, detrital components are rare and mostly consist of scattered silt-sized quartz and carbonate grains. Modern varve formation commenced about a century ago and is favoured by anthropogenic eutrophication (Kienel et al., 2013). A prominent characteristic of the Holocene sediment profile is alternation of homogeneous and varved sediment intervals with various varve micro-facies types described by Dräger et al. (2017) (Fig. 3).
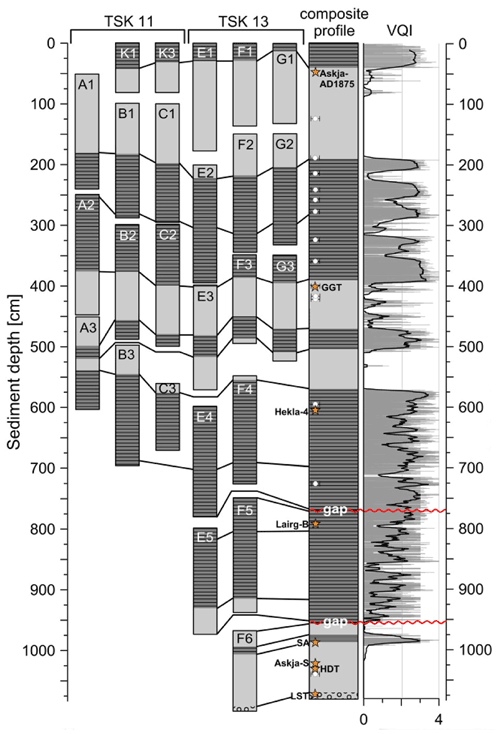
Figure 3Composite profile from 2011 and 2013 cores comprising the last ca. 6000 years (from Dräger et al., 2017). Indicated are varved and homogeneous sediment intervals and the degree of varve preservation (varve quality index (VQI): high values reflect well-preserved varves). Cryptotephra horizons are indicated. LST: Laacher See tephra; HDT: Hässeldalen tephra; SA: Saksunarvatn ash; GGT: Glen Garry tephra.
In addition to the long sediment profile from the deepest part of the lake basin, numerous short cores from the sediment surface of down to 150 cm depth have been obtained from all parts of the lake basin (Fig. 4). Their records provide detailed insight into the spatial propagation of the sediments. For most parts of the basin, sediment variations are small and only the onset of varve preservation varies with water depth (Dräger et al., 2019). In shallower water depths, varves start several decades later than in deeper water. This is due to the gradual expansion of anoxic conditions of the bottom waters. For example, at a water depth of 35 m varves have occurred only since 1980 CE; they began forming 60 years later than at 62 m water depth. At two locations in the northern and southwestern part of the basin, sediments appear very differently. Organic Holocene sediments are no more than 60 cm thick and deposited directly on top of glacial till. In the sediment seismic image these locations are clearly visible (Fig. 4) as black spots which indicate small morainic remnants within the subglacial valley that forms the lake basin.
2.2 Chronology
The age model for the sediment profile is based on varve counting, radiocarbon dating and tephrochronology. Varve counting is restricted to annually laminated intervals and provides robust information about sedimentation rates that are used to support dating of non-varved intervals. In addition, varve counting provides the link to the present since varve formation has occurred until today. Besides varve counting 28 radiocarbon dates from well-defined organic macro-remains are included in the age determination of the chronology. Of these dates, 14 are published in the age model covering the last 6000 years (Fig. 5; Dräger et al., 2017), while the other 14 radiocarbon dates in the age range between 6000 and 11 600 a cal BP are still unpublished.
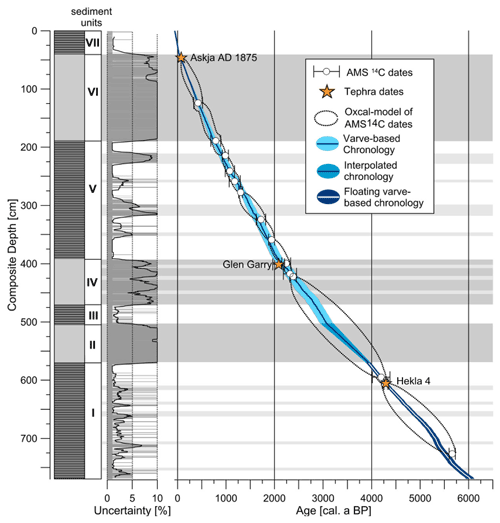
Figure 5Age model for the Tiefer See sediment profile of the last 6000 years from Dräger et al. (2017). Coloured bars indicate intervals of poor varve preservation or non-varved intervals.
Another seven anchor points for the chronology are provided by tephrochronology. Besides the 1–2 mm thick visible layer of the Saksunarvatn ash (10 210±35 a cal BP), six crypto-tephra horizons have been identified that can be assigned to known volcanic eruptions on Iceland: the early Holocene Hässeldalen and Askja-S tephras, the mid-Holocene Lairg-B (6683±45 a cal BP) and Hekla-4 (4293±43 a cal BP) tephras, the Glen Garry tephra (2088±122 a cal BP), and the historical Askja-AD1875 tephra. The latter is an important time marker for the end of the Little Ice Age and appears 4 decades before the onset of varve formation. The Hässeldalen and Askja-S tephras occur in non-varved sediments and thus form essential time markers for a robust early Holocene dating. Besides their importance for dating the Tiefer See sediments, these tephra findings allow precise synchronization with other sediment records in the circum-Baltic region (Wulf et al., 2016) and beyond. Another emerging tool for synchronising sediment records is 10Be analyses as a proxy for solar variability. In the sediments from Tiefer See three grand solar minima during the last 6000 years confirm the age model and provide time markers for synchronization (Czymzik et al., 2018).
Last but not least, an independent and precise determination of the onset of the Holocene has been achieved by pollen analyses (Martin Theuerkauf, personal communication, 2021).
2.3 Instrumental monitoring setup
Since 2012 permanent monitoring has been established in Tiefer See at the location from where the long Holocene sediment profile has been obtained (i.e the deepest part of the lake). At this position, weather and lake water parameters as well as sediment deposition at different water depths are continuously measured (Fig. 6). The monitoring setup includes an anchored platform with a weather station and profiler with a water probe measuring water and several lake water parameters down to a water depth of 55 m in 12 h intervals. Attached to this platform is a movable platform with a tripod used for water sampling at seven different water depths between 1 and 50 m, mainly for stable oxygen and deuterium isotopes in monthly intervals as well as for sampling surface cores once a year. A second pyramid-shaped platform with a profiler and water probe as backup is anchored at a short distance southwest of the main platform. Water measurements are carried out parallel to those with the water probe installed at the main platform. Weather and water monitoring is complemented by three different types of sediment traps collecting sediments at different time intervals and water depths: two four-cylinder traps at 12 m water depth below the thermocline and near the lake bottom at 50 m water depth integrate sedimentation over a month. A sequential sediment trap near the lake bottom collects sediments biweekly, and a two-cylinder trap installed as a backup nearby accumulates sediments in half-year intervals. Regular sediment analyses from all traps except the backup trap included measurements of total carbon, organic carbon and nitrogen as well as stable isotopes (δ13Corg, δ15N, δ13Ccarb and δ18Ocarb). The high-resolution data on biochemical calcite precipitation in the lake have been used, for example, to calibrate remote sensing data of regional calcite formation in northeastern German lakes (Heine et al., 2017). The monitoring concept is flexible and can be easily extended for limited time intervals in case it is needed for more specific research questions about, for example, processes of cyanobacteria deposition in the sediments (Nwosu et al., 2021a, b, c). More technical details about the monitoring devices installed can be found at https://www.gfz-potsdam.de/en/section/climate-dynamics-and-landscape-evolution/infrastructure/lake-sediment-monitoring (last access: 4 August 2022).
2.4 Modern varve formation
The primary goal of the lake monitoring is to decipher the processes of varve formation including seasonal effects and, in particular, those external forcing mechanisms that mainly control the depositional processes. Before starting the sediment monitoring at Tiefer See it was known that calcite varves have been and preserved for about a century (Kienel et al., 2013). Calcite varves are the common varve type in annually laminated lakes in formerly glaciated areas of the southern Baltic lowlands and are described as light–dark couplets with the light layer representing endogenic calcite formation in spring and summer (Tylmann et al., 2013). Calcite precipitation occurs in the epilimnion of the lake because, there, light conditions favour photosynthetic activity of phytoplankton (i.e. diatom blooms) which exploits CO2 from the epilimnion, leading to carbonate saturation (e.g. Kelts and Hsü, 1978). This process is clearly reflected in the sedimentation processes in Tiefer See, starting with a distinct diatom bloom during spring warming in April and/or in presently rare cases of longer winter ice cover immediately after the ice break-up (Fig. 7; Kienel et al., 2017; Roeser et al., 2021a). As reported from other calcite varves, we also observe a gradation of calcite crystal sizes from large crystals in the initial phase of calcite formation. The transition between the diatom and calcite sublayers is not sharp, confirming the causal relation between algal growth and calcite formation. Comparing calcite amounts in sediment traps from the epilimnion and hypolimnion reveals that sometimes parts of the calcite formed in the epilimnion are dissolved during settling through the water column (Roeser et al., 2021a).
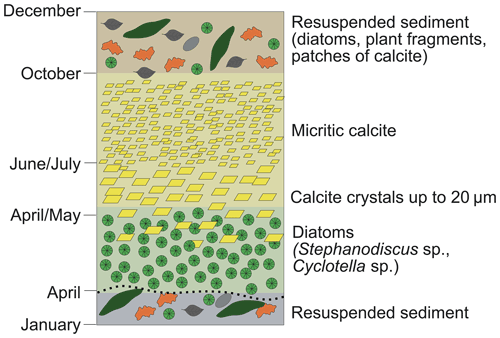
Figure 7Schematic presentation of sedimentation during the course of the year and division of three seasonal sublayers (modified from Roeser et al., 2021a).
The majority of calcite is deposited in May and June although from July to September some calcite formation may continue. In October, sediment deposition shifts from almost pure calcite to a mixture of different components including diatom frustules (more epiphytic taxa than planktonic); patches of calcite; plant fragments; and, rarely, scattered silt-sized detrital mineral grains. These sediments predominantly originate from shallower parts of the lake basin and are re-suspended by wind and wave activity during autumn mixing of the water column. In most years of the monitoring period, no longer period of ice cover occurred so that sediment re-suspension continued during winter. In summary, three distinct phases of sedimentation processes can be allocated to seasonal changes in the lake (Fig. 8): (1) diatom bloom, (2) calcite formation and (3) re-suspension. These phases form discrete seasonal sublayers in the sediment record that can be clearly identified through micro-facies analyses. In contrast, the macroscopic image reveals only light–dark couplets because the re-suspension and diatom layer together appear as a dark sublayer. This has to be considered for interpreting varve thickness data because very different processes determine the individual sublayers. In particular, wind and wave activity play a more important role for sedimentation than has previously been assumed. Besides the regular recurrence of the three phases of sedimentation, we observe substantial year-to-year variability in the quantity of sediments formed in each season. This variability appears to be largely driven by the timing and strength of water circulation (e.g. spring mixing) and wind-driven wave activity. Consequently, wind conditions and strength of water stratification seem to constitute a more important factor for sedimentation rate than temperature and precipitation.
The observatory Hinnensee (Fig. 9a), located in the Serrahn part (SE) of Müritz National Park, was selected for several reasons. The landscape around Hinnensee is mostly flat, but the northern shores are an exception to this, as they are formed by steep slopes with altitudinal differences of up to 50 m within short distances (Kaiser et al., 2014), resulting in dry/wet micro-site conditions distant from/near to the groundwater at the uphill/downhill locations. The hydrological setting of the groundwater-fed lake Hinnensee is relatively simple. Its lake level is governed solely by water intake from precipitation plus surface runoff from the surrounding slopes and groundwater, as well as water loss from evapotranspiration. The lake has a narrow ratio of lake vs. catchment area and therefore shows immediate and significant lake level responses after precipitation events (Van der Maaten et al., 2015). Large parts of the Hinnensee surroundings in Serrahn are covered by forest. About 75 % of the Serrahn area is covered by Scots pine (Pinus sylvestris L.), sessile oak (Quercus petraea Liebl.) and European beech (Fagus sylvatica L.) forests of different age cohorts. The forest at the northern shore of Hinnensee is an old-growth oak–beech–pine forest. Nature in Serrahn has been protected since the 18th century for hunting and was declared a wilderness conservation area in 1961 (Nationalparkamt Müritz, 2006). It became part of Müritz National Park in 1990, and the park's Serrahn subsection has been designated a UNESCO World Heritage Site for its old-growth beech forest, which contains up to 400-year-old beech stands and over 250-year-old pines (Spiess, 2015). This status as a protected region for such a long period ensures natural or at least near-natural growth conditions.
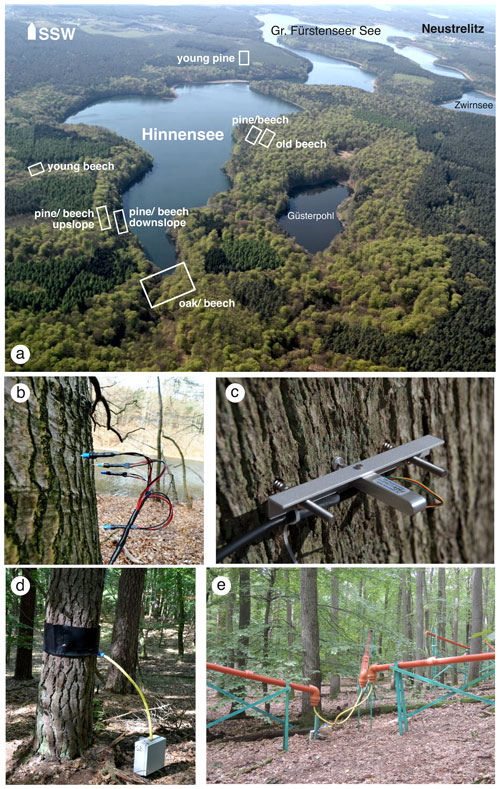
Figure 9View of the tree-ring and hydrological observatory Hinnensee with its forest monitoring plots (a). (b) Monitoring of tree transpiration with sap flow sensors and (c) half-hourly stem growth with dendrometers. Forest hydrological monitoring with (among others) (d) a stemflow collector and (e) canopy throughfall collectors.
3.1 Instrumental monitoring setup
The tree-ring observatory Hinnensee comprises instrumental monitoring of the weather conditions including tree-crown throughfall, tree stemflow and leaf wetness, soil moisture and temperature, lake and groundwater levels, tree stem diameter growth, and sap flow (estimate for tree transpiration) (Fig. 9b–e). The measurements are conducted every 30 min.
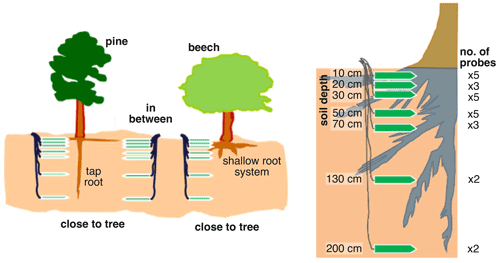
Figure 10Setup of soil moisture monitoring. Several probes are installed at various rooting depths in between and close to different trees and tree species with different rooting depths.
Soil moisture variability is recorded with 450 sensors at seven different depths between 10 and 200 cm at 15 sites (Fig. 10).
3.2 Selected results
The soil moisture data at the Hinnensee site show the dynamics for the period from 2014–2018 (Fig. 11). The extreme year of 2018 is particularly characterized by above-average wetness until April, followed by strong and continuous drought, which, unlike in normal years, was hardly interrupted by the usual occasional summer rains. From the beginning of June, the minimum of soil moisture prevailed. This minimum was also reached in previous years but always for short periods only. As the water provided by the summer rainfall events is normally absorbed directly by the forest stand, it was to be expected that the trees would suffer particularly from this deficiency in 2018. This should apply first of all to trees that are located further up the slope (right column) as these locations far from the groundwater are drier than directly at the lake, where the distance to the groundwater is only between 0.5 and 4 m (left column).
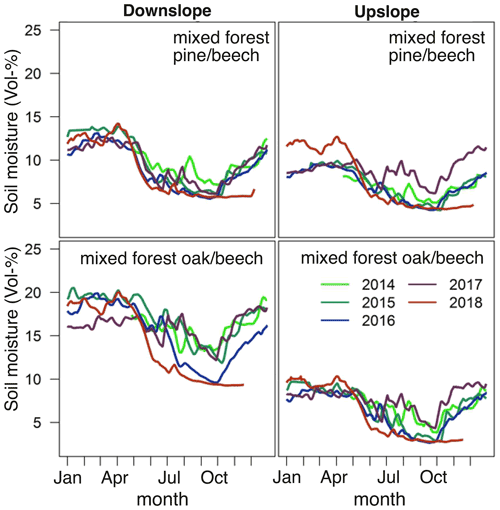
Figure 11Annual mean soil moisture dynamics for the years 2014–2018 at four monitoring locations around Hinnensee, Müritz National Park.
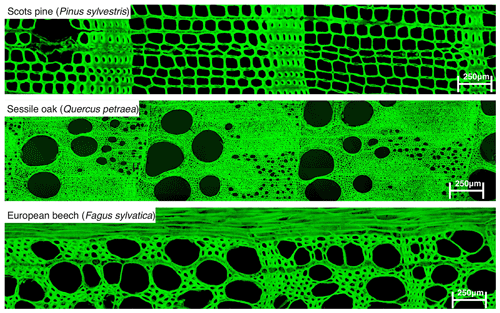
Figure 12Microscopic images of stem wood of monitored tree species Pinus sylvestris (pine), Quercus petraea (oak) and Fagus sylvatica (beech).
Not only do the monitored tree species have very different wood anatomical structures (Fig. 12), but also they have different reactions to the extreme conditions of the year 2018.
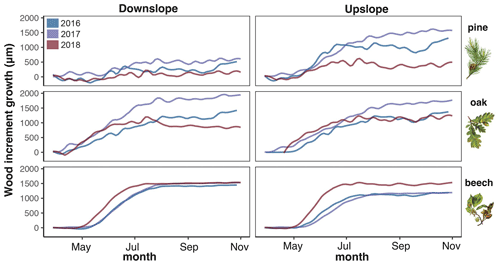
Figure 13Annual course of wood increment growth of pine, oak and beech (from top to bottom) at site Hinnensee (2016 to 2018). Other years are not shown for better clarity.
Figure 13 displays the annual incremental course of wood accumulation of beech, oak and pine that was continuously measured with point dendrometers (Fig. 9c) throughout the growing season of 2018.
While beech is surprising with astonishingly good growth, especially in locations far from groundwater, the other two tree species show strong declines in growth. This is surprising because beech trees are considered to have less resistance to drought (Scharnweber et al., 2011). The main explanation is that the beech trees generally grow faster in the early part of a growing season and also they were still able to benefit from the residual moisture in the soil. Thus, the beech trees had formed a rather normal annual tree ring before the extreme drought set in at the beginning of June 2018. Despite having formed a normal tree ring, the dendrometer data in Fig. 13 clearly show that the growth of the beech trees began and ended earlier in the year 2018. However, the growth curve is steeper than in previous years; i.e. despite a shorter growing period, the beech trees were able to produce a normal annual ring due to their faster growth. For oaks and pines, growth starts at similar times to beeches, with earlier growth for oaks and slower growth for pines and correspondingly narrower annual rings in 2018. For all three tree species it remains to be seen how extremely dry summers or winter-to-spring periods will affect the long-term course of growth. It can be assumed that the drought stress will lead to an undersupply of trees. As a result, the lack of reserves will lead to a delay in growth because after hibernation the trees first have to form new photosynthesis products before wood cells of the following annual rings can then be produced. However, the tree damage caused by the extreme year 2018 was limited and probably mitigated by the soil moisture still available at the beginning of the growing period.
3.3 Using tree rings for reconstructions
Several climatological and environmental factors can influence tree growth. Hence, it is a great challenge, from a methodological point of view, to evaluate to what extent and by which processes climatic changes are mirrored in the tree-ring archive, particularly from temperate regions, where the majority of people live. Novel methods and proxies (quantitative wood cell structure analyses and stable isotopes of carbon, oxygen and hydrogen) have been developed to cope with this challenge and help to better assess present-day forest health. The monitoring of climate signal transfers from atmosphere and soil into tree rings at Hinnensee is of crucial importance to test, improve and verify these new approaches to enhance our ability to quantify past climatic changes, which is in return necessary to enable better prediction of climate change impacts. It is of particular importance to disentangle climate signals from the multiple local to regional environmental influences acting on the physical and chemical characteristics of tree rings.
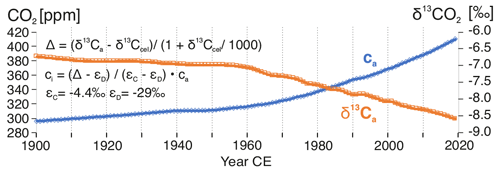
Figure 14Increase in atmospheric CO2 (ca) and corresponding δ13C since 1900 CE. Data taken from the compilation by Belmecheri and Lavergne (2020). Equations shown are simplifications of the model of carbon isotope fractionation during photosynthesis (Farquhar et al., 1982) from which retrospective estimates of leaf-internal CO2 (ci) concentrations from tree-ring δ13C (δ13Ctree) are calculated.
For example, the climate signatures of stable carbon and oxygen isotopes in tree rings of Scots pine (Pinus sylvestris L.) and sessile oak (Quercus petraea L.) in northeastern Germany do reflect regional hydrological signals in the high-frequency domain (year to year) well. However, long-term trends (decadal to multi-decadal) deviate strongly between oak and pine and carbon and oxygen isotopes. Well-known fractionations of carbon isotopes during photosynthetic uptake of atmospheric CO2 (ca; Fig. 14) into tree leaves through their pore openings (stomata) allow the retrospective assessment of leaf-internal CO2 concentrations (ci) from tree-ring δ13C data.
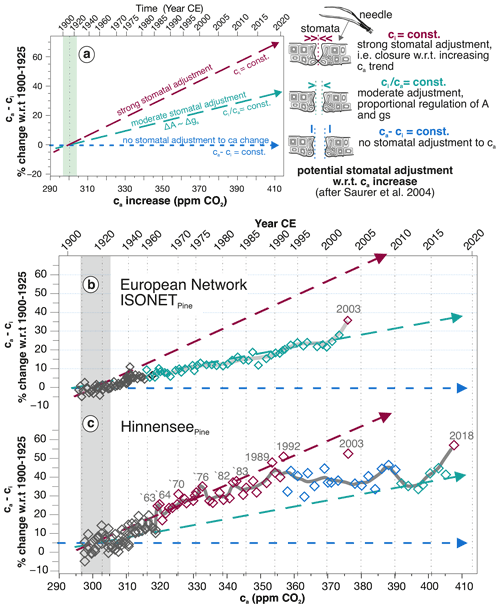
Figure 15Leaf stomatal response to atmospheric CO2 rise given as ca−ci (percent change with respect to 1900–1925) derived from δ13Ctree. (a) Three categories of potential stomatal adjustment: strong (ci is constant), moderate (ca=ca is constant) and no response (ca−ci is constant). A moderate response is ca increase is usually observed; hence photosynthetic CO2 assimilation (A) and stomatal conductance (gs) are regulated proportionally. (b) Average ca−ci of a network of pine stands across Europe (ISONET, 1900–2003); (c) ca−ci of pine from Hinnensee, indicating an episode of environmental stress from SO2 pollution between 1963–1992 and subsequent recovery, except for extreme drought years in 2003 and 2018. All dates plotted indicate drought years (graphs modified after Helle et al., 2022).
This helps to evaluate the response of forest trees in terms of trends in stomatal aperture and ci with regard to the rise in atmospheric CO2 concentrations (ca−ci; Fig. 15a). While increasing ca can stimulate photosynthesis and tree growth, it can also help to mitigate drought stress from climate warming as it allows the trees to reduce the apertures of the stomata for reducing transpiration and saving water while keeping ci and related photosynthesis rather constant.
Proxy and model analysis show that trees actually do a bit of both (Cernusak et al., 2019; Frank et al., 2015): since the beginning of the 20th century they have tended to close the stomata moderately with ca increase, allowing them to slightly constrain water loss by transpiration and also increase photosynthetic rates to a certain extent. This is expressed in a constant ratio as shown by a number of pine trees from a European tree network (Fig. 15b).
A strong response of stomatal closure relative to ca increase would correspond to a constant ci scenario, while no stomatal reaction to changing ca would be reflected in ca−ci giving a constant scenario (Fig. 15a) (Saurer et al., 2004). While the majority of European pines follow the moderate path of stomatal response to ca rise, pines from Hinnensee revealed a distinct period of strong stomatal reaction between 1963 to 1992 CE. This indicates an environmental stress on photosynthesis and points to potentially impaired tree vitality, although no external symptoms were reported.
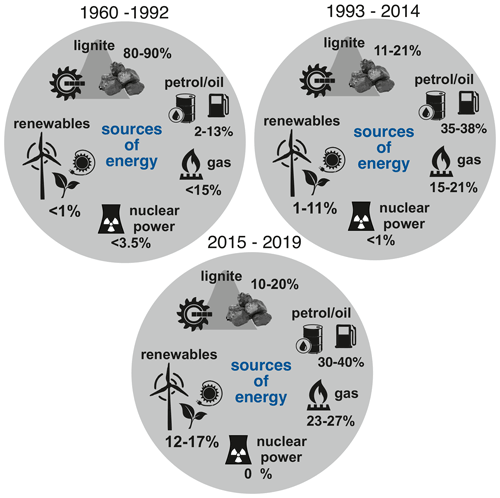
Figure 16Change in sources of primary energy in northeastern Germany (former GDR) (after AG Energiebilanzen e.V., 2020).
The reconstructed strong stomatal response can most likely be attributed to sulfur dioxide (SO2) air pollution. During German Democratic Republic (GDR) times, East Germany inflicted high stress on the environment by unfiltered and almost exclusive burning of sulfur-rich lignite in pursuit of independence in the energy sector (Fig. 16; Acker et al., 1998; AG Energiebilanzen e.V., 2020). SO2 pollution was pervasive in the brown-coal regions of Lusatia and central Germany around Leipzig with their large brown-coal power plants. However, the rural northeast German lowlands were considered to be only marginally affected by the heavy air pollutant emissions prevailing in certain regions south of Berlin until 1990.
Indeed, the forests of Müritz National Park showed no visible external symptoms of reduced forest health during the 1980s, the period of highest SO2 emissions. But, linking tree-ring δ13C-derived ci data to ca changes revealed a previously unknown and unexpected impact of air pollution in this area prevailing particularly from 1963 to 1992 (Fig. 15c). Interestingly, broadleaf oak trees from Hinnensee did not record any SO2 stress in their tree-ring isotope signature (not shown here). This difference between deciduous oak and evergreen pine can be attributed to the seasonality of emissions, which were high in winter and low during summer times without domestic heating and with reduced demand for electricity because of longer daylight periods. Apparently, critical thresholds were passed along with the seasonal changes in SO2 emissions. Whereas pine needles were damaged by high SO2 concentrations from enhanced lignite burning during winter or transition periods (autumn, spring), oak leaves were not affected due to low summer SO2 emissions causing no damage to the photosynthetic system. While this example shows that tree-ring δ13C is not a direct physical climate proxy and can be influenced by other atmospheric changes like CO2 rise or air pollution, tree-ring δ18O does contain a strong and direct hydrological signal averaged over several precipitation events and modified by tree transpiration, which depends on air humidity. Hence, time series of tree-ring δ18O can be used for lake level reconstructions as shown in Fig. 17 comparing the tree-ring δ18O chronology from pine at Hinnensee with instrumental lake level measurements of Gr. Fürstenseer See (Hinnensee is the northeastern section of Gr. Fürstenseer See; Fig. 9a).
The following can be highlighted:
-
Regional coal burning emissions produce non-climatic shifts in tree isotope records.
-
A previously undetected episode of reduced tree vitality (1963–1992) due to SO2 emissions has been identified by tree-ring δ13C (ca−ci) in evergreen pine from Müritz National Park. Deciduous oak was not affected due to seasonality in SO2 emissions.
-
Hydroclimate signals, e.g. lake level fluctuations, are well preserved in tree-ring δ18O of pine despite air pollution episodes.
-
Previous damage from a past SO2 pollution episode may have increased the vulnerability of pine compared to broadleaf oak to the most recent droughts (e.g. 2018 and 2019).
Tiefer See sediment record data are available at https://doi.org/10.1594/PANGAEA.862117 (Dräger et al., 2016). Lake sediment monitoring data are available at GFZ Data Services (https://doi.org/10.5880/GFZ.4.3.2020.003; Roeser et al., 2021b). General information on the TERENO Northeastern German Observatory is available at https://www.tereno.net/joomla/index.php/observatories/northeast-german-lowland-observatory (last access: 16 August 2022) and the TERENO TEODOOR portal (https://ddp.tereno.net/ddp/, last access: 16 August 2022). Soil moisture data are available at https://timeseries.gfz-potsdam.de (last access: 16 August 2022, login required). Tree-ring data and dendrometer data can be requested by contacting dendro52@gfz-potsdam.de. Dendrometer data will be also available at https://timeseries.gfz-potsdam.de but due to technical issues only after September 2022.
AB and TB designed the TERENO Northeastern German Observatory. AB is responsible for the lake monitoring and jointly wrote the manuscript together with IH, with contributions from MJS and GH. IH and GH are responsible for the dendrological monitoring, and TB is responsible for the hydrological monitoring at Hinnensee. BP provided stable isotope data from Tiefer See, and DB analysed dendrological data. BB, MK and SP carried out fieldwork at Tiefer See, including coring, data collection and quality control.
The contact author has declared that none of the authors has any competing interests.
Publisher's note: Copernicus Publications remains neutral with regard to jurisdictional claims in published maps and institutional affiliations.
We thank the Nature Park Nossentiner/Schwinzer Heide (Tiefer See) and the Müritz National Park (tree rings) for constant support of our work. Andreas Hendrich is acknowledged for the preparation of figures from Tiefer See.
Acker, A., Möller, D., Marquardt, W., Brüggemann, E., Wieprecht, W., Auel, R., and Kalaß, D.: Atmospheric research program for studying changing emission patterns after German unification, Atmos. Environ., 32, 3435–3443, https://doi.org/10.1016/S1352-2310(98)00041-7, 1998.
AG Energiebilanzen e.V.: Auswertungstabellen zur Energiebilanz für die Bundesrepublik Deutschland, Daten für die Jahre von 1990 bis 2019, BDEW Bundesverband der Energie- und Wasserwirtschaft e.V., https://ag-energiebilanzen.de/daten-und-fakten/auswertungstabellen/ (last access: 20 November 2021), 2020.
Belmecheri, S. and Lavergne, A.: Compiled records of atmospheric CO2 concentrations and stable carbon isotopes to reconstruct climate and derive plant ecophysiological indices from tree rings, Dendrochronologia, 63, 125748, https://doi.org/10.1016/j.dendro.2020.125748, 2020.
Cernusak, L. A., Haverd, V., Brendel, O., Le Thiec, D., Guehl, J. M., and Cuntz, M.: Robust Response of Terrestrial Plants to Rising CO2, Trends Plant Sci., 24, 578–586, 2019.
Czymzik, M., Muscheler, R., Adolphi, F., Mekhaldi, F., Dräger, N., Ott, F., Słowinski, M., Błaszkiewicz, M., Aldahan, A., Possnert, G., and Brauer, A.: Synchronizing 10Be in two varved lake sediment records to IntCal13 14C during three grand solar minima, Clim. Past, 14, 687–696, https://doi.org/10.5194/cp-14-687-2018, 2018.
Dräger, N., Theuerkauf, M., Szeroczynska, K., Wulf, S., Tjallingii, R., Plessen, B., Kienel, U., and Brauer, A.: Lake Tiefer See varve microfacies, bulk geochemistry, µ-XRF, vegetation openness and Cladocera record, PANGAEA [data set], https://doi.org/10.1594/PANGAEA.862117, 2016.
Dräger, N., Theuerkauf, M., Szeroczynska, K., Wulf, S., Tjallingii, R., Plessen, B., Kienel, U., and Brauer, A.: A varve micro-facies and varve preservation record of climate change and human impact for the last 6000 years at Lake Tiefer See (NE Germany), Holocene, 27, 450–464, https://doi.org/10.1177/0959683616660173, 2017.
Dräger, N., Plessen, B., Kienel, U., Słowiński, M., Ramisch, A., Pinkerneil, S., and Brauer, A.: Relation of δ13C of sedimentary organic matter and varve preservation in the sediment record of Lake Tiefer See (NE Germany), J. Paleolimnol., 62, 181–194, https://doi.org/10.1007/s10933-019-00084-2, 2019.
Farquhar, G. D., O'Leary, M. H., and Berry, J. A.: On the relationship between carbon isotope discrimination and the intercellular carbon dioxide concentration in leaves, Aust. J. Plant Physiol., 9, 121–137, 1982.
Frank, D. C., Poulter, B., Saurer, M., et al.: Water-use efficiency and transpiration across European forests during the Anthropocene, Nat. Clim. Change, 5, 579–583, https://doi.org/10.1038/nclimate2614, 2015.
Hardt, J. and Böse, M.: The timing of the Weichselian Pomeranian ice marginal position south of the Baltic Sea: A critical review of morphological and geochronological results, Quatern. Int., 478, 51–58, 2018.
Helle, G., Armanetti, C., Balanzategui, D., Heinrich, I., Schleser, G. H. S., Schürheck, L., Schwabe, M., and Wagner, R.: Tree-ring stable isotopes from Scots pine reveal past episode of air pollution in rural NE Germany, Sci. Total Environ., in review, 2022.
Heine, I., Brauer, A., Heim, B., Itzerott, S., Kasprzak, P., Kienel, U., and Kleinschmit, B.: Monitoring of calcite precipitation in hardwater lakes with multi-spectral remote sensing archives, Water, 9, 15, https://doi.org/10.3390/w9010015, 2017.
Heinrich, I., Balanzategui, D., Bens, O., Blasch, G., Blume, T., Böttcher, F., Borg, E., Brademann, B., Brauer, A., Conrad, C., Dietze, E., Dräger, N., Fiener, P., Gerke, H. H., Güntner, A., Heine, I., Helle, G., Herbrich, M., Harfenmeister, K., Heußner, K.-U., Hohmann, C., Itzerott, S., Jurasinski, G., Kaiser, K., Kapple, C., Koebsch, F., Liebner, S., Lischeid, G., Merz, B., Missling, K. D., Morgner, M., Pinkerneil, S., Plessen, B., Raab, T., Ruhtz, T., Sachs, T., Sommer, M., Spengler, D., Stender, V., Stüve, P., and Wilken, F.: Interdisciplinary Geo-ecological Research across Time Scales in the Northeast German Lowland Observatory (TERENO-NE), Vadose Zone J., 17, 180116, https://doi.org/10.2136/vzj2018.06.0116, 2018.
Heinrich, I., Balanzategui, D., Bens, O., Blume, T., Brauer, A., Dietze, E., Gottschalk, P., Güntner, A., Harfenmeister, K., Helle, G., Hohmann, C., Itzerott, S., Kaiser, K., Liebner, S., Merz, B., Pinkerneil, S., Plessen, B., Sachs, T., Schwab, M. J., Spengler, D., Vallentin, C., and Wille, C.: Regionale Auswirkungen des Globalen Wandels: Der Extremsommer 2018 in Nordostdeutschland, System Erde, 9, 38–47, https://doi.org/10.2312/GFZ.syserde.09.01.6, 2019.
Kaiser, K., Küster, M., Fülling, A., Theuerkauf, M., Dietze, E., Graventein, H., Koch, P. J., Bens, O., and Brauer, A.: Littoral landforms and pedosedimentary sequences indicating late Holocene lake-level changes in northern central Europe – a case study from northeastern Germany, Geomorphology, 216, 58–78, https://doi.org/10.1016/j.geomorph.2014.03.025, 2014.
Kelts, K. and Hsü, K.: Freshwater carbonate sedimentation, in: Lakes: Chemistry, Geology, Physics, edited by: Lerman, A., Springer, Berlin, 295–323, https://doi.org/10.1007/978-1-4757-1152-3_9, 1978.
Kienel, U., Dulski, P., Ott, F., Lorenz, S., and Brauer, A.: Recently induced anoxia leading to the preservation of seasonal laminae in two NE-German lakes, J. Paleolimnol., 50, 535–544, 2013.
Kienel, U., Kirillin, G., Brademann, B., Plessen, B., Lampe, R., and Brauer, A.: Effects of spring warming and mixing duration on diatom deposition in the deep Tiefer See, NE Germany, J. Paleolimnol., 57, 37–49, https://doi.org/10.1007/s10933-016-9925-z, 2017.
Lüthgens, C., Böse, M., and Preusser, F.: Age of the Pomeranian ice-marginal position in northeastern Germany determined by Optically Stimulated Luminescence (OSL) dating of glaciofluvial sediments, Boreas, 40, 598–615, 2011.
Nantke, C. K. M., Brauer, A., Frings, P. J., Czymzik, M., Hübener, T., Stadmark, J., Dellwig, O., Roeser, P., and Conley, D. J.: Human influence on the continental Si budget during the last 4300 years: δ30Sidiatom in varved lake sediments (Tiefer See, NE Germany), Quaternary Sci. Rev., 258, 106869, https://doi.org/10.1016/j.quascirev.2021.106869, 2021.
Nationalparkamt Müritz: Forschung und Monitoring 1990–2006, Schriftenreihe über Forschungsergebnisse im Müritz-Nationalpark, Vol. 1, https://www.mueritz-nationalpark.de/service/fach-informationen (last access: 8 August 2022), 2006.
Nwosu, E. C., Brauer, A., Kaiser, J., Horn, F., Wagner, D., and Liebner, S.: Evaluating sedimentary DNA for tracing changes in cyanobacteria dynamics from sediments spanning the last 350 years of Lake Tiefer See, NE Germany, J. Paleolimnol., 66, 279–296, https://doi.org/10.1007/s10933-021-00206-9, 2021a.
Nwosu, E. C., Roeser, P., Yang, S., Ganzert, L., Dellwig, O., Pinkerneil, S., Brauer, A., Dittmann, E., Wagner, D., and Liebner, S.: From Water into Sediment – Tracing Freshwater Cyanobacteria via DNA Analyses, Microorganisms, 9, 1778, https://doi.org/10.3390/microorganisms9081778, 2021b.
Nwosu, E. C., Roeser, P., Yang, S., Pinkerneil, S. Dittmann, E., Brauer, A., Wagner, D., and Liebner, S.: Species-Level Spatio-Temporal Dynamics of Cyanobacteria in a Hard-Water Temperate Lake in the Southern Baltics, Front. Microbiol., 12, 761259, https://doi.org/10.3389/fmicb.2021.761259, 2021c.
Roeser, P., Dräger, N., Brykała, D., Ott, F., Pinkerneil, S., Gierszewski, P., Lindemann, C., Plessen, B., Brademann, B., Kaszubski, M., Fojutowski, M., Schwab, M. J., Słowiński, M., Błaszkiewicz, M., and Brauer, A.: Advances in understanding formation of calcite varves: new insights from a dual lake monitoring approach, Boreas, 50, 419–440, https://doi.org/10.1111/bor.12506, 2021a.
Roeser, P., Dräger, N., Brykała, D., Ott, F., Pinkerneil, S., Gierszewski, P., Lindemann, C., Plessen, B., Brademann, B., Kaszubski, M., Fojutowski, M., Schwab, M. J., Słowiński, M., Błaszkiewicz, M., and Brauer, A.: TERENO Monitoring data from Lake Tiefer See and Lake Czechowskie (2012–2017), GFZ Data Services [data set], https://doi.org/10.5880/GFZ.4.3.2020.003, 2021.
Saurer, M., Siegwolf, R. T. W., and Schweingruber, F. H.: Carbon isotope discrimination indicates improving water-use efficiency of trees in northern Eurasia over the last 100 years, Glob. Change Biol., 10, 2109–2120, 2004.
Scharnweber, T., Manthey, M., Criegee, C., Bauwe, A., Schröder, C., and Wilmking, M.: Drought matters – Declining precipitation influences growth of Fagus sylvatica L. and Quercus robur L. in north-eastern Germany, Forest Ecol. Manage. 262, 947–961, https://doi.org/10.1016/j.foreco.2011.05.026, 2011.
Spieß, H. J.: Geschichtlicher Abriss des Naturschutzes im Serrahner Gebiet, in: Neue Beiträge zum Naturraum und zur Landschaftsgeschichte im Teilgebiet Serrahn des Müritz-Nationalparks, edited by: Kaiser, K., Kobel, J., Küster, M., and Schwabe, M., Forschung und Monitoring, Geozon Science Media, 4, ISBN 978-3-941971-11-0, 2015.
Theuerkauf, M., Dräger, N., Kienel, U., Kuparinen, A., and Brauer, A.: Effects of changes in land management practices on pollen productivity of open vegetation during the last century derived from varved lake sediments, The Holocene, 25, 733–744, https://doi.org/10.1177/0959683614567881, 2015.
Theuerkauf, M., Blume, T., Brauer, A., Dräger, N., Feldens, P., Kaiser, K., Kappler, C., Kästner, F., Lorenz, S., and Schult, M.: Holocene lake-level evolution of Lake Tiefer See, NE Germany, caused by climate and land cover changes, Boreas, 51, 299–316, https://doi.org/10.1111/bor.12561, 2022.
Tylmann, W., Zolitschka, B., Enters, D., and Ohlendorf, C.: Laminated lake sediments in northeast Poland: distribution preconditions for formation and potential for paleoenvironmental investigation, J. Paleolimnol., 50, 487–503, 2013.
Uścinowicz, S.: Southern Baltic area during the last deglaciation, Geol. Q., 43, 137–148, 1999.
Van der Maaten, E., van der Maaten-Theunissen, M., Buras, A., Scharnweber, T., Simard, S., Kaiser, K., Lorenz, S., and Wilmking, M.: Can We Use Tree Rings of Black Alder to Reconstruct Lake Levels? A Case Study for the Mecklenburg Lake District, Northeastern Germany, PLOS ONE, 10, e0137054, https://doi.org/10.1371/journal.pone.0137054, 2015.
Wulf, S., Dräger, N., Ott, F., Serb, J., Appelt, O., Gudmundsdottir, E., van den Bogaard, C., Słowinski, M., Błaszkiewicz, M., and Brauer, A.: Holocene tephrostratigraphy of varved sediment records from Lakes Tiefer See (NE Germany) and Czechowskie (N Poland), Quaternary Sci. Rev., 132, 1–14, 2016.
Zacharias, S., Bogena, H., Samaniego, L., Mauder, M., Fuß, R., Pütz, T., Frenzel, M., Schwank, M., Baessler, C., Butterbach-Bahl, K., Bens, O., Borg, E., Brauer, A., Dietrich, P., Hajnsek, I., Helle, G., Kiese, R., Kunstmann, H., Klotz, S., Munch, J. C., Papen, H., Priesack, E., Schmid, H. P., Steinbrecher, R., Rosenbaum, U., Teutsch, G., and Vereecken, H.: A network of terrestrial environmental observatories in Germany, Vadose Zone J., 10, 955–973, 2011.
Zebisch, M., Grothmann, T., Schröter, D., Hasse, C., Fritsch, U., and Cramer, W.: Climate change in Germany: Vulnerability and adaptation of climate sensitive sectors, Research Report 201 41 253, UBA-FB 000844/e, Umweltbundesamt, Dessau-Roßlau, Germany, ISSN 1611-8855, 2005.